In the year 1998, Paul Anastas and John C. Warner presented a set of guidelines for green chemistry. The twelve principles of green chemistry have offered a range of options for minimizing the negative effects that chemical manufacturing has on the environment and human health.
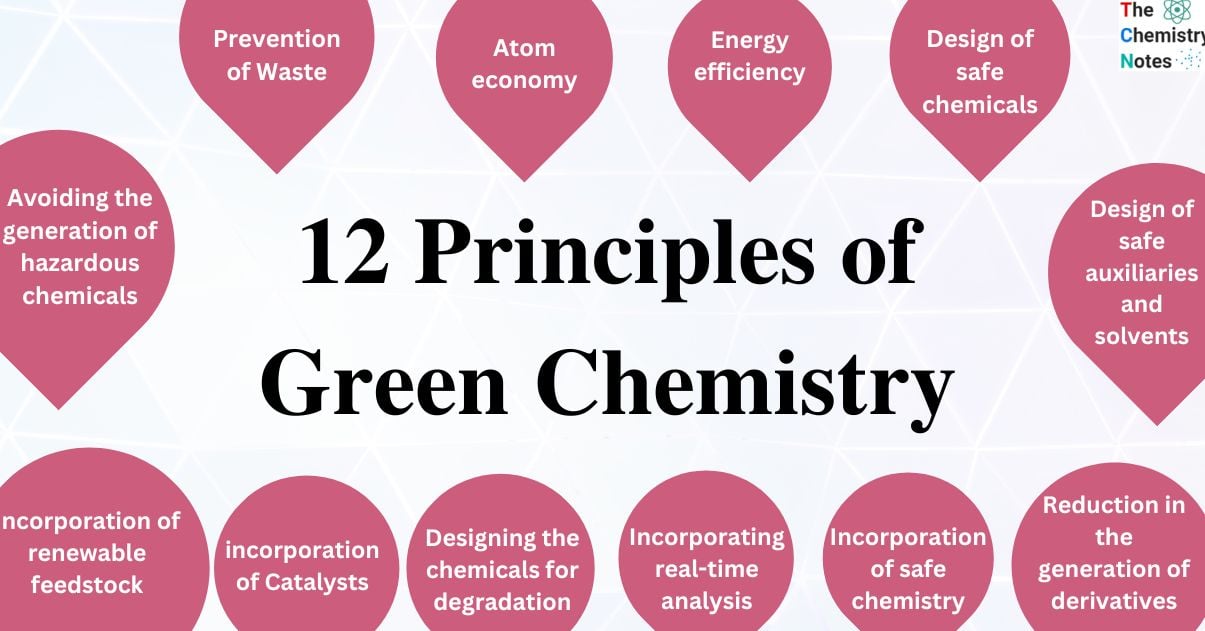
It also offers chances for the research and development of green technology. The 12 Principles of green chemistry includes:
- Prevention of Waste
- Atom economy
- Avoiding the generation of hazardous chemicals
- The design of safe chemicals
- Design of safe auxiliaries and solvents
- Energy efficiency
- Incorporation of renewable feedstock
- Reduction in the generation of derivatives
- Incorporation of Catalysts
- Designing the chemicals for degradation
- Incorporating real-time analysis
- Incorporation of safe chemistry for the prevention of accidents
Let us discuss in brief about the 12 enlisted principles of green chemistry.
Interesting Science Videos
Prevention of Waste
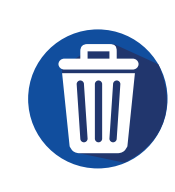
It is better to prevent waste than to treat or clean up waste after its formation
This is one of the most widely used guidelines in process optimization; it describes chemists’ ability to redesign chemical transformations to reduce the generation of hazardous waste as an important step toward pollution prevention. The hazards associated with waste storage, transportation, and treatment could be reduced by preventing waste generation. This principle is simple to grasp and apply, and examples from both industry and academia have demonstrated its significance, relevance, and feasibility. This green chemistry pillar remains valid; however, we must consider it in a broader context, shifting from a limited interpretation of waste based on quantity to a universal approach to dealing with the topic “waste”:
- We must consider the multidimensional nature of waste.
- We must shift away from the “quantity of waste per quantity of product” principle and toward the “quantity of waste generated per function provided by the product.” In this context, we must strive to improve both product quality and functionality.
- When considering a product’s entire life cycle, we must address the fact that not only does the manufacturing process generate waste, but “end-of-life waste” accumulates after the product’s life span or consumption. This includes both the conversion of previously considered waste materials into valuable products and their recyclability.
Moving toward “zero-waste production” and “waste prevention” in general entails modernizing industrial processes through clean manufacturing techniques. However, simply not producing the desired product would be the most auspicious strategy for reducing waste generation.
An alternative approach is to prevent the product from degrading into hazardous waste, for example, by making plastics biodegradable instead of highly recalcitrant petrochemical plastics. According to these ideas, we need to fundamentally reconsider our understanding of waste as a hazardous material.
Atom economy
Atom economy is a concept developed in the early 1990s to assess the effectiveness of chemical conversions element by element. The concept of “atom economy” is based on the ratio of the total mass of atoms in the target product to the total mass of atoms in the starting materials, which is analogous to well-established yield calculations. Planning such chemical transformations to maximize the integration of all materials used in the process into the final product, resulting in as few wasted atoms as possible, is one option for reducing waste generation. As a result, choosing chemical conversion routes that incorporate the majority of starting materials into desired products is more efficient and contributes to waste reduction.
This concept is now widely used in new routes to generate various organic compounds, such as those used in biomedical and pharmaceutical applications.
The development of green chemistry is one factor hastening the incorporation of pollution prevention into industrial manufacturing processes. Another definition states that chemical synthesis methods should be designed to maximize the incorporation of various potentially hazardous materials, such as spinning precarious waste with cement and sand to produce improved paste used in construction applications or incorporating radioactive wastes as immobilizing material to produce a safe stabilized form of waste. Similarly, combining recycled poly(ethylene terephthalate) (PET) waste with cement paste demonstrates a viable strategy for immobilizing hazardous wastes.
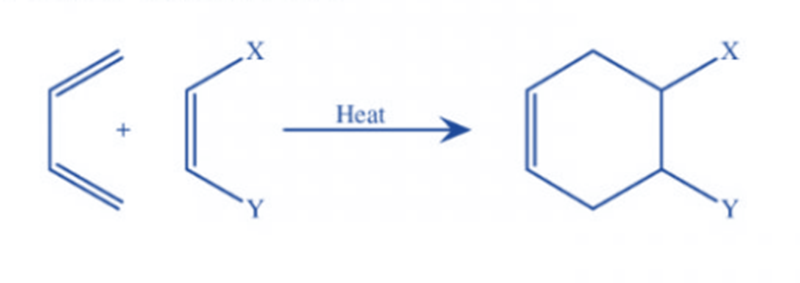
Avoiding the generation of hazardous chemicals
The principles of green chemistry what matter in synthetic organic chemistry: achieving a successful chemical transformation in a new way. Researchers have demonstrated a direct relationship between toxicity and the hazards associated with chemical reactions and the matrix of matter. The chemistry behind a process contributes to a chemical synthesis have a significant impact on the sustainability and green chemistry. An exception is made when a molecule is purposefully produced with the intent of displaying toxicity and/or biological activity. This scenario can be found in the case of various molecules synthesized for pharmaceutical or agricultural applications.
The selection of compounds used to increase the efficacy of chemical transformations is a critical point in the process. Chemists should pay special attention to the choice of materials to be placed in reaction vessels. It is simple to disregard all other materials and focus all efforts solely on the chemosynthetic pathway. However, disregarding all other matters present in a production process results in a high price to be paid. Because chemists occasionally produce hazardous molecules, the principle is dedicated to the design of molecules that are intrinsically safer.
The design of safe chemicals
Chemical products should be designed to perform their intended function while minimizing their toxicity. New products can be created that are inherently safer while still being highly effective for the intended application. Direct incorporation of radioactive spent liquid scintillation waste into cement combined with clay materials. This is considered an added value in the immobilization of hazardous organic wastes in very cheap materials and natural clay. Hence, it produce a safe stabilized product that is easy to handle, transform, and dispose of.
Design of safe auxiliaries and solvents
This principle encourages the use of less hazardous solvents and auxiliaries. It is concerned with substances that do not directly contribute to the structure but are required for the chemical reaction. Most organic compound reactions occur in liquid environments, where the solvent acts in a variety of ways. It can improve contact between reactants, stabilize or destabilize generated intermediates, or influence transition states. Furthermore, the solvent used governs the selection of appropriate downstream and regeneration processes, as well as recycling or discarding techniques. Developing innovative concepts for the substitution of volatile organic solvents has become a major challenge in green chemistry.
A green solvent should meet a variety of criteria, including low toxicity, nonflammability, nonmutagenicity, nonvolatility, and widespread availability. Furthermore, these green solvents must be inexpensive, easy to handle, and recyclable. Extensive recovery of microbial polyhydroxyalkanoate (PHA) biopolymers, which are typical intracellular storage materials, from biomass is provided as an example. For this extraction process, which is carried out with hazardous halogenated solvents is increasingly turning to less harmful greener solvents.
Energy efficiency
Typically, energy is used to improve human life in significant ways. Traditional energy sources such as coal, oil, and gas are in short supply, and their combustion emits greenhouse gases. Moving toward renewable energy and designing for energy efficiency are both required for continuous improvement of life quality. Designing more efficient processes by selecting the most appropriate technologies and unit operations must occur concurrently with the selection of appropriate energy sources. Using an electric motor with energy sources generated by the sun and wind is more environmentally friendly than using fossil fuels. The most important questions for engineers and designers to help society use energy more effectively are how energy is converted to useful forms and where it is lost.
As a result, green chemistry entails reducing energy losses such as mechanical friction, fluid drag, and unwanted heat transfer by improving the layout and insulation of a refrigerator or designing lighter vehicles with improved aerodynamic characteristics and lower rolling resistance.
Furthermore, when developing a new production process, the effect of the geographical location of production plants must be considered. Bioplastics show energy production technologies and the available energy have an impact on the ecological footprint of a new process.
Incorporation of renewable feedstock
When technically and economically feasible, raw material or feedstock should be renewable rather than depleting. Using renewable resources, such as microbial or plant biomass is a viable option for sustainably producing functional bioproducts.
The current global dynamic of these developments does provide cause for optimism for the future. A way to convert raw wastes into a mortar composite stabilized material is a great application of green chemistry. When switching from fossil to renewable feedstocks, it is important to remember that using renewable resources expands the process concept by incorporating resource provision, transportation, storage, and other logistics aspects into the process design.
However, such a shift in feedstock provision results in a fundamental shift in the structure of processes, technologies used, and economic concepts of industry and society.
Reduction in the generation of derivatives
Many processes could be designed to reduce the use of additional reagents and the waste that results from them. It is necessary to synthesize a derivative of a compound with groups not required as final product but facilitate the synthesis steps. However, these derivatives introduce atoms that are not incorporated into the final product but end up as waste. This contradicts the atom economy principle. Chemists are currently devoting research efforts to finding alternatives to protecting groups for many reactions that have traditionally required them.
Incorporation of Catalysts
Catalysis is a chemical reaction that is facilitated or accelerated by a catalyst. Catalysts are essential in our modern industrial economy, environmental stewardship, and all biological processes.
Recently, the synthesis of nano-catalysts of specific sizes and shapes has been developed. This allows the easy movement of materials in the reacting phase and control over the morphology of nanostructures to tailor their physical and chemical properties. Nano-catalyst systems with a paramagnetic core enable selective chemical transformations with high product yield and ease of catalyst recovery.
When discussing catalysis, it is important to mention biocatalysis; thus, the use of enzymes as highly selective and active catalysts. Not only do enzymes perform the desired reactions under mild temperature and pressure conditions. Furthermore, they are predestined to drive reactions of renewable materials which enable reactions that are impossible to achieve with traditional catalysts. Furthermore, biocatalysts, whether free or immobilized, are increasingly being used for bioremediation, and thus the removal of pollutants from the environment.
Microwave-driven chemical reactions for organic chemistry are an emerging field in catalysis that can drastically reduce reaction times from days to seconds. This technique has evolved over the last few decades from a laboratory prank to a well-established technology that is now widely used in industry.
Designing the chemicals for degradation
One of the most important goals of green chemistry is to maximize output while minimizing unwanted byproducts. It is reported that the design of products and processes that have a low impact on humans and the environment. Such as the creation of a sustainable mortar composite that could be considered a value-added product suitable for various applications as an inert matrix for immobilization of some low and intermediate levels of radioactive waste, decorative tiles, building bricks, and light concrete, is being reported. In this case, highly reactive hydroxyl radicals react with the organic moieties of the spinning fiber wastes by subtracting hydrogen ions or adding to the unsaturated site to produce organic radicals that are easily oxidized by oxygen. As a result, the only end products of the degradation process were carbon dioxide and water.
Incorporating real-time analysis
With advances in chemistry, the production of numerous toxic chemicals has become a serious environmental problem. Pollution prevention professionals are familiar with one of the fundamental concepts of green chemistry. For many years, the call has been made to use less hazardous materials in chemical formulations. As a result, green chemistry aims to eliminate the use and generation of hazardous substances by designing better chemical material manufacturing processes with minimal waste production through real-time monitoring of running processes. As a result, timely intervention is possible before waste or toxins are generated.
Incorporation of safe chemistry for the prevention of accidents
It is critical to avoid highly reactive chemicals, which could potentially cause accidents during the reaction. The substance and form of a substance used in a chemical process should be chosen in such a way that the possibility of chemical accidents, such as toxin releases, explosions, and fire formation, is minimized. Water, for example, could accidentally cause an explosion by flowing into a tank containing methyl isocyanate gas, releasing a large amount of methyl isocyanate into the surrounding area. Alkali metals contain other well-known materials that undergo often disastrous reactions with water. If an alternative reaction that did not use this reagent had been developed, the risk of explosion, even death, would have been reduced.
Intrinsically safe chemistry can also be performed in flow mode, using tubular microreactors with tiny reaction channels. Such flow chemistry approaches drastically reduce reaction volume, reaction time, and catalyst requirement, and intensify processes by increasing space/time yield. Hence, it opens new process windows in terms of extreme temperature and pressure conditions and even allows for the safe execution of highly dangerous reactions. Furthermore, the use of flow chemistry in microreactors demonstrates a strategy for overcoming the traditional drawbacks of microwave-driven processes.
Conclusion: Principles of Green Chemistry
Though these 12 principles of green principle are useful when designing new chemicals, many newer reactions yet offer this level of optimization flexibility. It can be difficult to apply green chemistry principles when designing all chemical reactions, especially in a laboratory setting. It is our responsibility to design reactions that are both safe and sustainable. With this in mind, applying the 12 principles of green chemistry should become second nature to us.
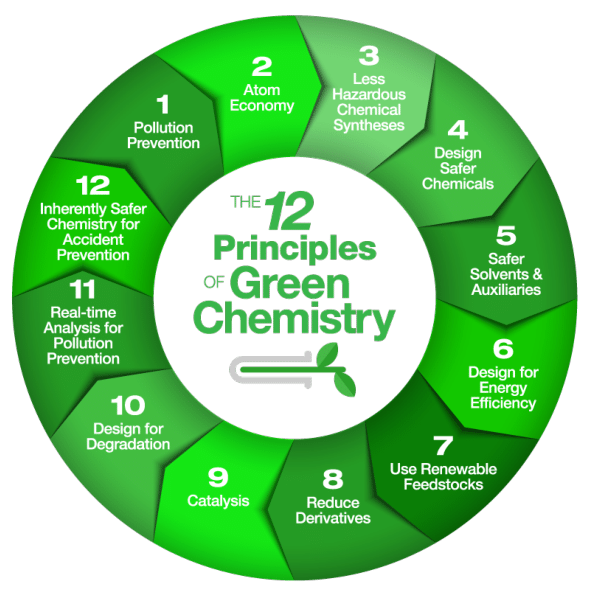
References
- Saleh, H. E. M. , & Koller, M. (2018). Introductory Chapter: Principles of Green Chemistry. In H. E. M. Saleh, & M. Koller (Eds.), Green Chemistry. IntechOpen. https://doi.org/10.5772/intechopen.71191
- Anastas, P., & Eghbali, N. (2010). Green chemistry: principles and practice. Chemical Society Reviews, 39(1), 301-312.
- Sawin, J. L., Sverrisson, F., Seyboth, K., Adib, R., Murdock, H. E., & Lins, C. (2014). Renewables 2014 global status report. Paris: REN21 Secretariat REN21.
- https://greenchemistry.yale.edu/about/principles-green-chemistry
- https://greenchemistry-toolkit.org/wp-content/uploads/2020/07/GC-4-Day_Training-Day-2-02-The-12-Principles-of-Green-Chemistry-April-2018-LU.
- https://www.e-education.psu.edu/eme807/node/534
- Eskander SB, Bayoumi TA, Saleh HM. Leaching behavior of cement-natural clay composite incorporating real spent radioactive liquid scintillator. Progress in Nuclear Energy. 2013;67:1-6
- Bayoumi TA, Saleh HM, Eskander SB. Solidification of hot real radioactive liquid scintillator waste using cement-clay composite. Monatshefte fur Chemie – Chemical Monthly. 2013;144(12):1751-1758
- Kerton FM. Alternative Solvents for Green Chemistry. Clark J: RSC Green Chemistry Series, Series Editors; 2009
- Hamed, I., Özogul, F., & Regenstein, J. M. (2016). Industrial applications of crustacean by-products (chitin, chitosan, and chitooligosaccharides): A review. Trends in Food Science & Technology, 48, 40-50.
- Hoffmann, R. W. (2006). Protecting-group-free synthesis. Synthesis, 2006(21), 3531-3541.