Atomic absorption spectrophotometry is a widely used analytical technique that involves the measurement of the absorption of electromagnetic radiation by atoms in the gas phase. Spectrophotometry is a scientific technique that involves the determination of the concentration of elements present in a liquid sample by measuring the amount of energy absorbed from specific wavelengths of light, typically ranging from 190 to 900 nm.
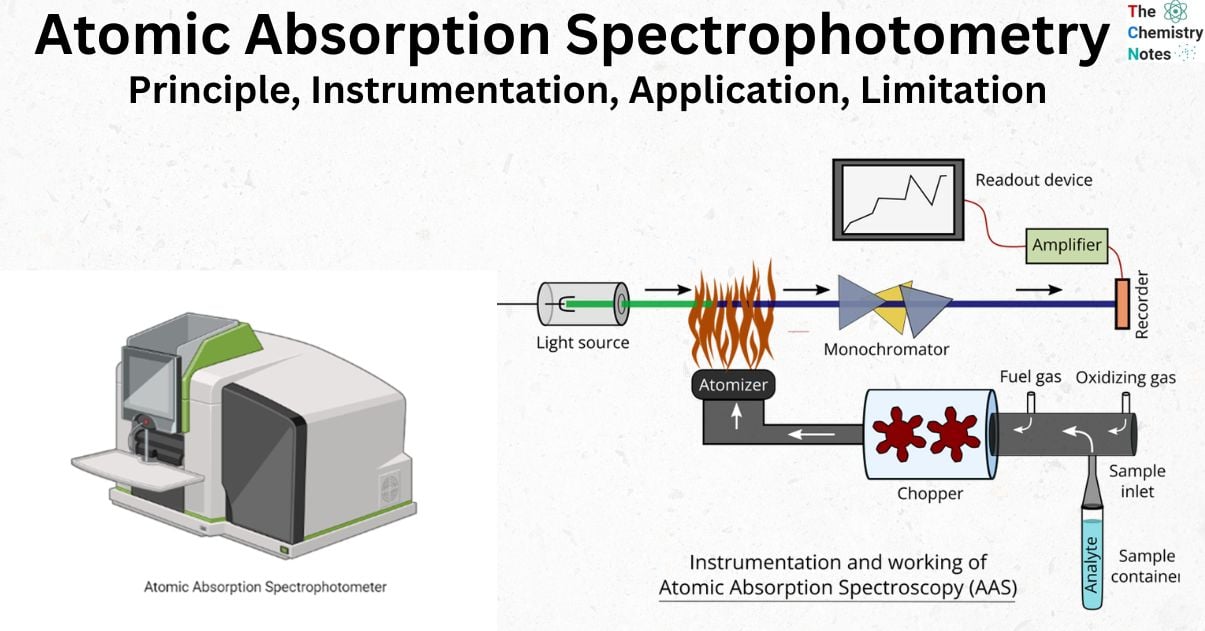
Atomic absorption spectrophotometers are commonly equipped with a flame burner that serves to vaporize the sample, which is typically a hollow cathode lamp. Additionally, these instruments include a monochromator and a photon detector.
This technique has extensive utility in the examination of trace metals present in a variety of samples, including soils, bodies of water such as lakes, rivers, and oceans, as well as potable water sources, pharmaceuticals, food and beverage products, geological and mineralogical specimens, petroleum derivatives, biological fluids and samples, and forensic investigations.
Interesting Science Videos
What is atomic absorption spectrophotometry?
The analytical technique of atomic absorption spectrophotometry is known for its high precision. This technique is employed to quantify the concentration of metallic elements within the sample. This methodology is considered to be among the most effective approaches for identifying minute quantities of a sample, such as those present at levels of one part per billion (ppb). The process includes the assimilation of radiant energy, typically ultraviolet and visible, by a collection of free atoms. The specimen employed is in a gaseous phase.
The absorption of light of a particular wavelength by an atom leads to the excitation of electrons from their ground state to the excited state. The quantity of energy absorbed per mole is constant and can be applied for both qualitative and quantitative assessments.
Atomic absorption spectrophotometry is a technique utilized for the identification and quantification of elements present in solid or liquid samples. This method involves the utilization of specific wavelengths of electromagnetic radiation emitted from a light source to detect the elements of interest. The differential absorption of wavelengths by individual elements is determined by measuring their absorbance against established standards. The Atomic Absorption Spectroscopy technique takes advantage of the distinct radiation wavelengths that are selectively absorbed by individual atoms.
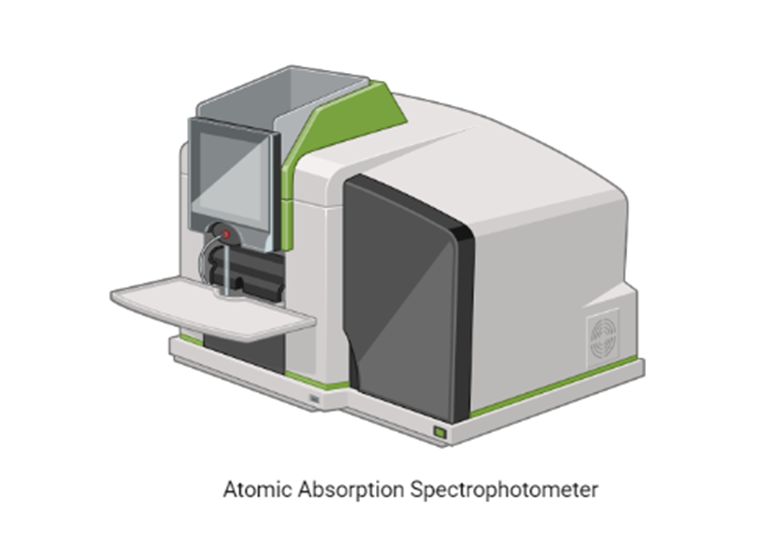
In simple terms, the Atomic Spectrophotometry depends on the fundamental capacity of atoms to either absorb or emit light. Atomic absorption spectrophotometry involves heating the atoms to a temperature that is sufficient to liberate them from solvents and prevent the formation of salts, while avoiding the excitation of electrons to higher energy levels. This is typically achieved through the use of a flame or graphite tube. The unbound atomic entities, possessing electrons in their lowest energy level, are poised to assimilate radiation of precise energy that would elevate the electrons to a higher electronic state.
The concentration of a specific atom in a given sample can be determined by measuring the amount of light it absorbs. This is due to the inverse relationship between the concentration of the atom and the amount of light that reaches the detector.
Principle of Atomic absorption spectrophotometry
The atoms comprising the analyte exhibit a constant and unaltered quantity of electrons. When a flame containing a particular atom is exposed to light of a specific wavelength, the electrons located in distinct energy levels, also referred to as orbitals, undergo excitation to higher energy levels by absorbing a particular wavelength. The relationship between the quantity of ground-state atoms present in the flame and the degree of absorption is directly proportional.
The lowest energy state, commonly referred to as the ground state, exhibits greater stability in comparison to the higher energy states, also known as excited states. The electrons exhibit a spontaneous tendency to return to their ground state. It emits an equivalent quantity of radiant energy. The phenomenon under discussion is commonly referred to as fluorescence. Atomic emission spectroscopy employs fluorescence as an approach.
As an example, upon absorption of a 589nm wavelength, the sodium (Na) atom undergoes excitation of its outermost electrons to higher energy levels. Subsequently, it returns to its initial or ground state. It is important to note that the emission of the 589nm wavelength remains consistent during the process of de-excitation.
The Boltzmann equation provides an explanation for the population of ground and excited states.
N1 is the number of atoms in the excited state.
No is the number of atoms in the ground state.
g1/go is the ratio of statistical weights for ground and excited states.
ΔE is the energy of excitation (=h∨).
k = Boltzmann constant.
Instrumentation of atomic absorption spectrophotometry
The basic instrumentation of atomic absorption spectroscopy is illustrated below:
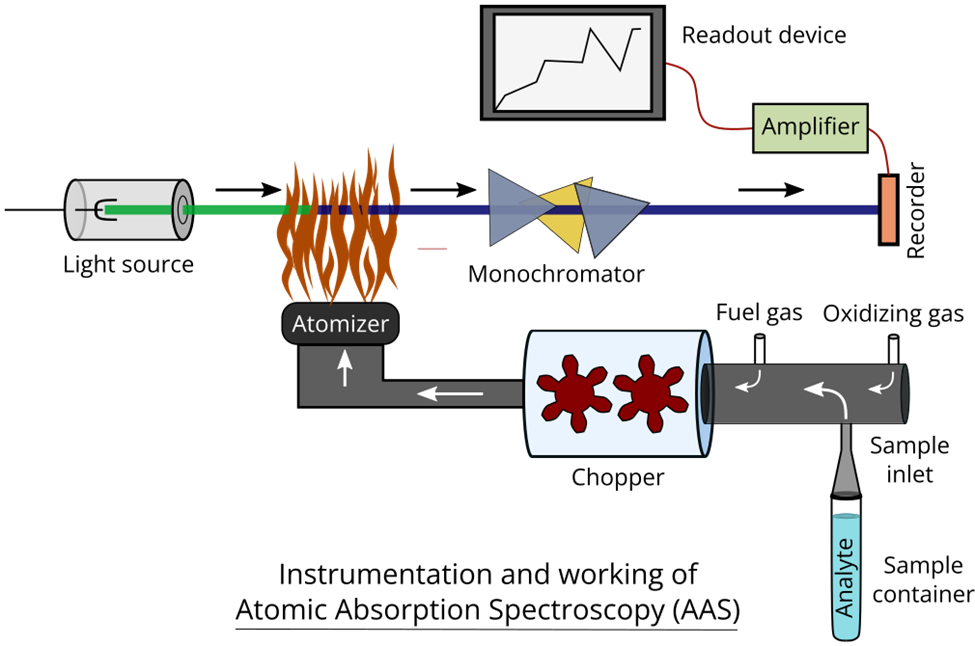
Light source: The light source employed in this study is a hollow cathode lamp. The apparatus comprises a cylindrical cathode that is hollow and composed of the identical element that is being examined, as implied by its terminology. Tungsten is the material constituting the anode. The anode and cathode are contained within a glass tube that consists of a quartz window.
The glass enclosure is charged with inactive gases, namely Argon and Neon, at a decreased atmospheric pressure ranging from 1 to 5 torr. Upon application of a high voltage (ranging from 300 to 500 V) to the electrodes, the noble gases situated at the anode undergo ionization. The ions generated as a consequence are propelled toward the cathode. Upon collision with the cathode surface, the high-velocity ions induce sputtering of certain metal atoms. The excitation of electrons to higher energy levels occurs when metals are vaporized. The reason for this phenomenon can be attributed to the persistent interaction with gas ions possessing greater energy levels.
Chopper: A chopper is a rotary device positioned within a hollow cathode lamp and a flame. The process eliminates macroscopic droplets and facilitates the entry of droplets with homogeneous dimensions into the combustion zone. The lamp’s continuous light is modulated into a pulsating form. The pulsating current within the photocell is amplified and recorded without any known interference.
- Sample introduction system: This apparatus is utilized for the purpose of transferring samples to the atomizer. The ideal scenario involves the absence of any interference, complete independence from the sample type, and universal applicability across all atomizers. Pneumatic nebulizers are a frequently employed means of introducing solutions. The solution is nebulized through a capillary tube by means of a compressed air jet, which also serves to aspirate and nebulize the nebulization gas.
- Nebulizers: Concentric nebulizers and angular or crossflow nebulizers are the two commonly employed nebulizers.
Atomizer: The process of atomization can be executed through two distinct methods, namely, by utilizing a flame or a furnace. Atomic absorption spectroscopy employs heat energy to transform metallic elements into atomic dissociated vapor. Precise temperature regulation is crucial for the successful conversion of atomic vapor. At elevated temperatures, the process of ionization can occur in atoms. The fuel and oxidant gases are introduced into a mixing chamber, which subsequently traverses baffles before reaching the burner. The instrument of absorption spectrophotometry generates a flame in the form of a ribbon. The specimen is introduced into the mixing chamber via aspiration.
Monochromators: A monochromator is an optical instrument that selectively transmits a narrow range of wavelengths of light or other forms of radiation from a broader spectrum of wavelengths. The Atomic Absorption Spectrophotometer (AAS) instrument facilitates the absorption of energy by its constituent atoms, which subsequently leads to the emission of radiation. A monochromator can be utilized to isolate a desired band of lines by transmitting a narrow band.
There are two types of monochromators: prism and diffraction gratings. The dispersion exhibited by a grating is comparatively more homogeneous in nature than the dispersion produced by a prism. The grating exhibits an extended range of wavelength resolution, thereby enabling its sustained performance over a prolonged period.
Detectors: The process of converting light originating from a monochromator into a simplified electrical signal can be accomplished through the use of a detector. Typically, a photomultiplier tube serves as the detector in the instrument utilized for atomic absorption spectrophotometry. A detector has the capability to be adjusted to react to a particular wavelength or frequency.
Amplifiers: The detectors’ output is transmitted to amplifiers, which proceed to increase the current’s magnitude by multiple orders of magnitude.
Readout devices: Various types of readout systems are employed in atomic absorption spectroscopy, including meters, chart recorders, and digital display meters. Hard copies can be produced through the use of chart recorders, printers, or plotters.
Working process for atomic absorption spectrophotometry
Typically, for the purpose of conducting atomic absorption spectrometry analysis, it is recommended that the samples be in the form of a solution. The following are the procedural steps involved in the analysis.
- The transformation of a given sample into a solution form.
- The process involves making a blank solution.
- Preparation of a series of standard solutions.
- Establishing instrumental parameters.
- Measure the light absorption of solutions of known concentration.
- A calibration curve was constructed by plotting the concentration of the sample against its corresponding absorbance value.
- Determine the level of light absorption exhibited by the solution sample.
- The determination of the concentration of an unknown element is derived through the utilization of a calibration curve.
Atomic absorption spectrophotometry flame
An atomic absorption spectrometry flame was generated by employing a fuel and an oxidant. Commonly employed fuels for flame generation include natural gas, propane, butane, hydrogen, and acetylene. In atomic absorption spectrophotometry, the process of flame formation for oxidation purposes involves the utilization of various oxidants such as air, oxygen, nitrous oxide, and a combination of nitrous oxide and acetylene.
The table below provides an overview of different types of flames along with their respective maximum temperatures.
Fuel | Air oxidant | Oxygen oxidant | Nitrous oxide oxidant |
Hydrogen | 2100 °C | 2780 °C | – |
Acetylene | 2200 °C | 3050 °C | 2955 °C |
Propane | 1950 °C | 1950 °C | – |
Low temperatures were employed for metals such as copper (Cu), lead (Pb), zinc (Zn), and cadmium (Cd). These metallic elements exhibit a high vapor pressure and readily undergo sublimation at relatively low temperatures.
Certain metallic elements exhibit low volatility and resistance to atomization or vaporization. Consequently, a significant degree of heat is required to achieve the vaporization of these particular metals. Elevated temperatures can be achieved through the utilization of an oxidizing agent in conjunction with fuel gas during atomic absorption spectroscopy. An oxyacetylene flame was employed to perform elemental analysis of aluminum, titanium, and rare earth elements using an atomic absorption spectrophotometer.
Quantitative analysis in atomic absorption spectrophotometry
The process of quantifying elements involves the utilization of calibration curves for plotting. To construct a linear calibration curve of absorbance versus concentration, a minimum of four standards and a solution with unknown concentration are necessary. In the context of absorbance measurements, it is imperative to ensure that the instrument zero is calibrated against a blank solution, which could be either distilled water or a solution with comparable composition to the sample being tested, prior to taking any readings.
Standards are typically evaluated in ascending order of concentration. Following the measurement of a solution, the flame is rinsed out with distilled water to eliminate any residual traces of the previous solution prior to the analysis of the subsequent solution. It is recommended to conduct a minimum of two to three distinct measurements and subsequently calculate their mean value.
Interferences
The occurrence of interference is a phenomenon that results in alterations in the intensity of the analyte. It reduces the degree of light absorption.
AAS interferences can be classified into two distinct categories: spectral and non-spectral interferences.
- The phenomenon of spectral interference primarily arises from the absorption caused by a neighboring atomic line or species, as well as a molecular absorbance band in proximity to the spectral line of a specific element under investigation. One potential solution to this problem involves selecting an alternative analyte line and utilizing background absorption measurements to accurately determine the atomic absorption through subtraction from the total measured absorption.
- Matrix interference is a type of non-spectral interference that arises when the physical properties of a sample, such as its viscosity or surface tension, differ from those of the standard. This discrepancy can lead to fluctuations in the rate of sample uptake, owing to alterations in nebulization efficiency. The reduction of interferences can be achieved by closely matching the matrix composition of the standard and sample.
- The phenomenon of chemical interference is observed in cases where a sample comprises of a particular species that, upon combination with an analyte, produces a molecule that is thermally stable and not completely disintegrated by the flame’s energy. The presence of interference can be inferred through the introduction of an excessive amount of a different element or compound.
- At elevated temperatures, the occurrence of ionization interference is more prevalent. The process of dissociation does not terminate upon the generation of atoms in their lowest energy state. Excessive energy in a flame has the potential to ionize atoms in their ground state by means of electron removal, resulting in a depletion of said ground state atoms. The introduction of an abundant quantity of a readily ionizable element into the flame generates a significant number of unbound electrons, which hinders the ionization of the substance being analyzed and eliminates any interference caused by ionization.
Background Correction
During the process of acquiring absorption spectra, the detector may detect signals originating from external particles present in the flame, resulting in interference from the background. The presence of peak broadening and spurious peaks in non-absorbing regions regardless, of the acquired spectrum remains indicative of the sample under investigation. The implementation of appropriate background correction methodologies can effectively mitigate these variances and amplify the signal originating from the analyte.
- Koirtyohann and Pickett invented the first automatic background technique utilizing a deuterium lamp and a hollow cathode lamp (single LS). The apparatus alternates between the deuterium continuum and the analyte source and subtracts background absorption from the hollow cathode lamp’s overall absorption. Deuterium’s ultraviolet wavelength limits the analyst’s wavelength range, hence this procedure has drawbacks.
- Smith and Hieftje used hollow cathode lamps with high and normal current pulsing to adjust the background. The normal current gives the entire absorbance, including interferences, and the high-current pulse gives the background. Only volatile items function with this method. The continual pulsing reduces the hollow cathode lamp’s lifetime and limits its usage to FAAS.
- LS GFAAS uses the Zeeman-effect background correction approach, which produces background versus sample data using an alternating magnetic field. It has limits when the sample contains another metal, such Ni or Fe, with spectral lines close to the analyte wavelength.
- HR-CS AAS’s software automatically selects corrective pixels on both sides of the analytical line that do not show absorption lines. Any radiation intensity change that affects all correction pixels will be automatically adjusted to the baseline. The signal output has light noise and continuous background adjusted. with contrast, background adjustments increase baseline noise by at least a factor two with LS AAS. This affects accuracy, LOD, and LOQ. The Resano et al. review article provides more details.
Application of atomic absorption spectrophotometry
- Mining and Geology: The determination of the elemental composition of minerals and rocks is a crucial factor in assessing the economic viability of mining operations in explored regions. After the extraction process, it is imperative to subject the extracted ores and minerals to compositional analysis to ensure optimal efficacy of the refining procedures. Trace metal analysis holds significant importance in the exploration of oil and water reservoirs.
- Environmental monitoring: The monitoring of environmental conditions with regard to trace metal pollution in industrial discharges, oceans, rivers, and lakes is a crucial aspect in determining the suitability of water for both commercial and potable purposes. The establishment of whether such samples fall within the safety limits prescribed by regulatory agencies is of paramount importance. The assessment and viability of a location for establishing commercial ventures are greatly influenced by environmental monitoring.
- Pharmaceuticals: The analysis of trace metals by the application of atomic absorption spectrophotometry is a crucial aspect in the development of formulations, determination of catalyst efficiency, and establishment of dosage limits. The majority of elements exhibit advantageous properties within specific recommended thresholds, however, surpassing said thresholds can result in detrimental consequences.
- Oil and petroleum: Refining is a necessary process for both edible oils and mineral oils prior to their consumption. The process of refining may encompass both catalytic refining and distillation. The absorption of metals in the course of these activities may result in decreased performance or pose potential risks to consumers. The analysis of trace metals in engine oil can offer valuable diagnostic insights into the degradation of engine components.
- Material development: The physical characteristics of materials, including but not limited to their hardness, brittleness, grain size, crystallinity, and amorphous nature, are subject to notable modification by their composition and the presence of trace metals. The analysis of trace metals via atomic absorption spectrometry can yield valuable insights into the performance characteristics of said materials.
- Food and beverages: Within the food and beverage sector, the utilization of this technique is prevalent for the purpose of evaluating the concentrations of diverse elements in wine, beer, and fruit-based beverages. Atomic absorption spectrometry is a technique that is employed for the identification of diverse forms of impurities present in food.
- Iron, manganese, copper, zinc, mercury, lead, and nickel can all be detected in human urine and blood.
- Forensic: It offers significant data on specimens, including stomach contents utilized for foodborne illness investigations, paint chips, fibers, and hair strands that are gathered from the location of a criminal incident.
- Agriculture: The technique of atomic absorption spectrophotometry is employed for the identification and quantification of metallic constituents, including but not limited to calcium, strontium, and cobalt, within soil samples. Furthermore, it is employed in the analysis of animal feed to determine the presence of various elements such as Zn, Cu, Mn, Ca, K, Na, among others. This technique is employed for the purpose of analyzing animal-derived fertilizer in order to ascertain the levels of various elements, including but not limited to Ca, Cu, Mg, and Fe.
- The implementation of this analysis is imperative in instances of heavy metal toxicity. Regular monitoring of poison levels in the patient’s blood is essential due to the potentially lethal nature of heavy metal poisoning.
- Before consuming or utilizing for irrigation purposes, it is important to analyze samples of groundwater and bore wells for metal content.
Advantages of atomic absorption spectrophotometry
- The precision of the results is high, with a typical range of 0.5% to 5%.
- As a means of detection, it exhibits remarkable sensitivity, quantifying at the level of parts per million (ppm).
- The distinctive light-absorbing properties of certain atoms enable them to be analyzed for specific elements.
- It may be used to measure the amounts of over 65 different elements.
- It’s an easy process that follows established, standard operating procedure
- When compared to other types of analysis, the cost is little.
- Atomic absorption spectrophotometry provides support to a diverse array of industries and sectors, encompassing but not limited to environmental, chemical, petrochemical, food and beverage, and pharmaceutical industries.
Disadvantages of atomic absorption spectrophotometry
Limited sensitivity
The instrument’s capacity is restricted to measuring a single element exclusively and its linearity is constrained.
References
- Filho HJI, Salazar RF, Capri M, Neto AC, de Alcantara MAK, Peixoto AL. State of the art trends in atomic absorption spectrometry. Intechopen. Published 2011.
- Walsh, A. Atomic Absorption Spectroscopy – stagnant or pregnant?
- H. H. Willard, L. L. Merritt, J. R. Dean, and F. A. Settle, Instrumental Methods of Analysis (7th Edition), CBS Publishers and Distributions, India, 1986.
- Vogel’s Textbook of Quantitative Chemical Analysis, 6th Edition, 2008.
- D.A. Skoog, F.J. Holler, and T.A. Nieman, Principle of Instrumental Analysis, 5th Edition.
- https://chem.libretexts.org/Bookshelves/Analytical_Chemistry/Physical_Methods_in_Chemistry_and_Nano_Science_(Barron)/01%3A_Elemental_Analysis/1.04%3A_Introduction_to_Atomic_Absorption_Spectroscopy
- https://chemistnotes.com/analytical_chemistry/atomic-absorption-spectroscopy-principle-instrumentation-and-7-reliable-application/
- https://www.priyamstudycentre.com/2021/11/atomic-absorption-spectroscopy.html
This is great . I did my research on Effects of Anionic and Cataionic pollutants on aquatic lives in Lake Victoria Kenya . I used Atomic spectroscopy for my elemental analysis and i hope one day i can publish this research paper