The first law of thermodynamics is one of the three fundamental laws of thermodynamics. It is derived from energy conservation but stated in a different and more useful manner for thermodynamics to include systems where heat transfer and work are the primary energy transfer methods.
Although the laws of thermodynamics appear simple to state, their implications are profound. According to the first law, if heat is recognized as a type of energy, then the total energy of a system plus its surroundings is conserved;
The total energy of the universe remains constant.
Interesting Science Videos
What is the First Law of Thermodynamics?
The first law of thermodynamics states that the total energy of an isolated system is constant. Energy can be transformed from one form to another but can neither be created nor destroyed.
The first law of thermodynamics was derived in the 19th century by Rudolf Clausius and William Thomson.
Nothing better illustrates the first law of thermodynamics than a gas (such as air or helium) trapped in a container with a tightly fitting movable piston (as seen below). We’ll assume the piston can move up and down, compressing or expanding the gas (but no gas is allowed to escape the container).
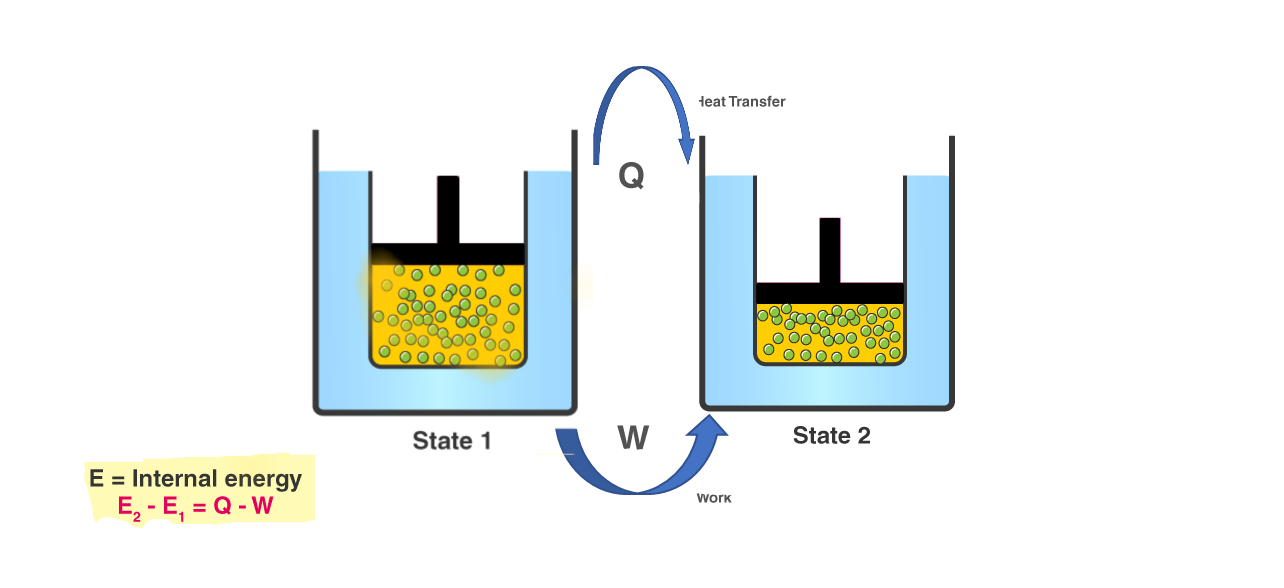
The “system” consists of the trapped gas molecules in the container. The molecules of gas have kinetic energy. The system’s internal energy U can be thought of as the sum of the kinetic energies of the individual gas molecules. As a result, as the gas’s temperature T rises, the gas molecules accelerate, and the gas’s internal energy U rises (which means, ∆U is positive). Similarly, as the gas’s temperature T falls, the gas molecules slow down, and its internal energy U falls (which means ∆U is negative).
It’s critical to remember that internal energy (U) and temperature (T) increase as gas molecule speeds increase since they’re just two ways of measuring the same thing: how much energy is in a system. Because the temperature and internal energy are directly proportional, when the internal energy doubles, so does the temperature. Similarly, if the temperature does not change, neither does the internal energy.
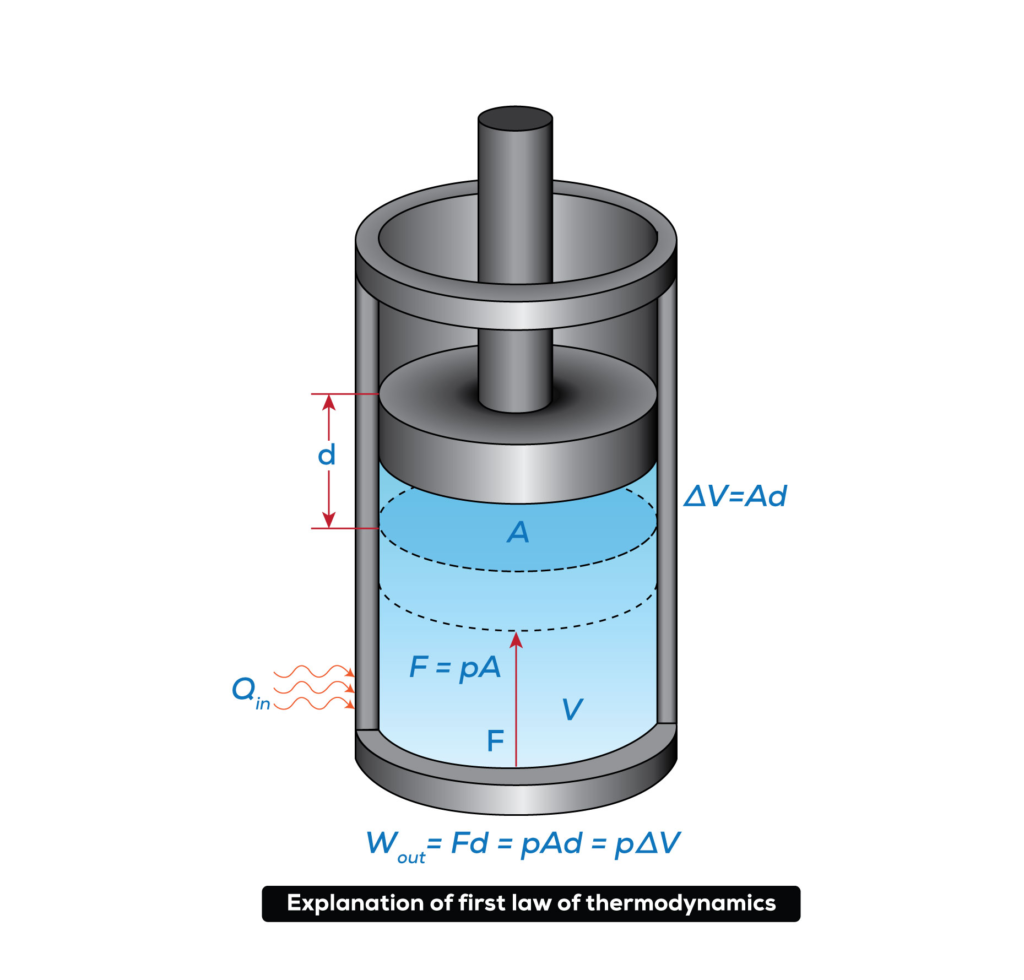
System and Surrounding
To gain a better understanding of how energy works in the universe, it is useful to divide it into two distinct parts. The first is the energy of a specific system, and the second is whatever energy was not included in the system, which we call “energy of surroundings”. Because these two parts equal the total energy of the universe, we can conclude that:
E (universe) = E (Surrounding) + E (System) (1)
Given that the total amount of energy in the universe does not change, one can set a change in energy of the system and surroundings to equal.
∆E (System) + ∆E (Surrounding) = 0 (2)
Rearranging Equation (2) leads to the;
∆E (System) = – ∆E (Surrounding) (3)
Equation 3 represents an essential tenet of energy conservation.
The underlying assumption is that any change in a system’s energy will cause an equal but opposite change in its surroundings. This basically sums up the First Law of Thermodynamics, which states that energy can neither be created nor destroyed.
First Law of Thermodynamics (Internal Energy)
Internal energy is a state variable in an equilibrium thermodynamic system. Heat transfer into the system minus work done by the system equals the internal energy difference between the two systems.
The energy of the universe does not change, according to the first law of thermodynamics. It can move between the system and its surroundings, but it cannot be created or destroyed. The law mostly concerns energy states caused by work and heat transmission. The idea of energy conservation is redefined.
The popular example of a heat engine helps to explain the meaning of the First Law. n a Heat engine, thermal energy is converted into mechanical energy, and the process is reversed. The vast majority of heat engines are open systems. The primary working principle of a heat engine is to take advantage of the numerous interactions between heat, pressure, and volume of a working fluid, which is typically a gas. It is not uncommon for gas to convert to a liquid and then back to a gas.
Now that energy conservation has been defined, one can investigate the various energies of a system. There are three types of energy in a system. These are kinetic (motion energy), potential (energy stored within a system as a result of placement or configuration), and internal (energy associated with electronic and intramolecular forces). As a result, the following equation can be given.
E (total) = KE + PE + U (4)
KE denotes kinetic energy, PE denotes potential energy, U denotes internal energy, and E (total) denotes total system energy. While all forms of energy are important, the internal energy, U, will receive most of the attention.
First Law of Thermodynamics (Heat)
The energy transferred by molecular motion and collisions due to a temperature difference is measured in joules as heat (Q). When the system is used as the reference, heat entering a system is considered positive, while heat exiting a system is considered negative.
First Law of Thermodynamics (Work)
The work (W) of a system, measured in joules, is the amount of energy transferred from one system to another or from one system to its surroundings. This is a broad category of mechanical work.
When a system of reference performs work, the work is defined as negative because energy is lost from the system of reference and consumed by an external system or the surroundings.
Work on the system of reference is defined as positive when energy is added to the system of reference and lost from an external system or the surroundings.
First Law of Thermodynamics Equation
As previously stated, U represents the energy associated with electronic and intramolecular forces. Despite the abundance of forces and interactions that may exist within a system, calculating its internal energy is nearly impossible. Instead, the change in a system’s U, U, must be measured. Two distinct variables influence the change in U of a system. These two variables are denoted by the letters q and w. The total amount of energy transferred to or from a system due to thermal contact is heat. The total amount of energy transferred to or from a system due to changes in the external parameters is work (volume, pressure). Applying this, the following equation can be given;
∆U = q + w (5)
If the change in U is negligible, Equation 5 can be changed to;
dU = dq + dw (6)
It should be noted in this equation that U is a state function and thus independent of pathways, whereas q and w are not.
However, the first law equation can be perplexing due to using two different sign conventions.
Sign Conventions
The change in energy of a system in physics, particularly when discussing heat engines, equals the heat flow in the system from the surroundings minus the work done by the system on the surroundings. The law’s equation can be written as follows:
ΔU = Q – W
In this equation, U represents the change in internal energy of a closed system, Q represents the heat supplied to the system, and W represents the amount of work the system does on its surroundings. This version of the law adheres to Clausius’ sign convention.
The IUPAC, on the other hand, follows Max Planck’s sign convention. Net energy transfer to a system is positive, while net energy transfer from a system is negative. The equation is then:
ΔU = Q + W
ΔU (Change in Internal Energy) | Q (Heat) | W (Work done on gas) |
(+) If Temperature increases | (+) If heat enters gas | (+) If gas is compressed |
(-) If Temperature decreases | (-) If heat exists gas | (-) If gas expands |
0 if Temperature is constant | 0 If no heat exchanged | 0 If volume is constant |
Applications of First Law of Thermodynamics
Adiabatic Process: An adiabatic process is one in which no heat can enter or exit the system.” Because there is no heat transfer across the system boundary in an adiabatic process, Q=0. According to the first law of thermodynamics:
E (internal) =Q +W
∴ ΔE (internal) = W [Since Q = 0]
The work done on the system increases the internal energy
Isothermal reaction: “The Isothermal process refers to a process in which the system’s temperature remains constant.” Because the temperature in the isothermal process remains constant, the internal energy of the gas must also remain constant, so:
E (internal) = Q +W
0 = Q + W
⇒ Q =-W
Limitations of the First Law of Thermodynamics
- The first law of thermodynamics has a limitation in that it says nothing about heat flow direction.
- It is not possible to undo the procedure. In reality, heat does not completely convert to labor. If we could convert all of the heat into work, we could move ships across the ocean by extracting heat from the water.
- It makes no distinction between spontaneous and non-spontaneous processes.
References
- R. Chang, “Physical Chemistry for the Chemical and Biological Sciences”, University Science Books, Sausalito, California (2000).
- https://chem.libretexts.org/Bookshelves/Physical_and_Theoretical_Chemistry_Textbook_Maps/Supplemental_Modules_(Physical_and_Theoretical_Chemistry)/Thermodynamics/The_Four_Laws_of_Thermodynamics/First_Law_of_Thermodynamics/First_Law_of_Thermodynamics
- https://www.studysmarter.co.uk/explanations/physics/engineering-physics/first-law-of-thermodynamics/
- https://www.thoughtco.com/first-law-of-thermodynamics-definition-604343
- https://www.sciencedirect.com/science/article/pii/B9780128145197000057
- Aston, J. and Fritz, J.J., Thermodynamics and Statistical Mechanics, John Wiley and Sons, Inc., New York, 1959.
- Atkins, P.W. and Julio de Paulo, Atkins’ Physical Chemistry, Oxford University Press, UK, Indian Edition 9, 2011.