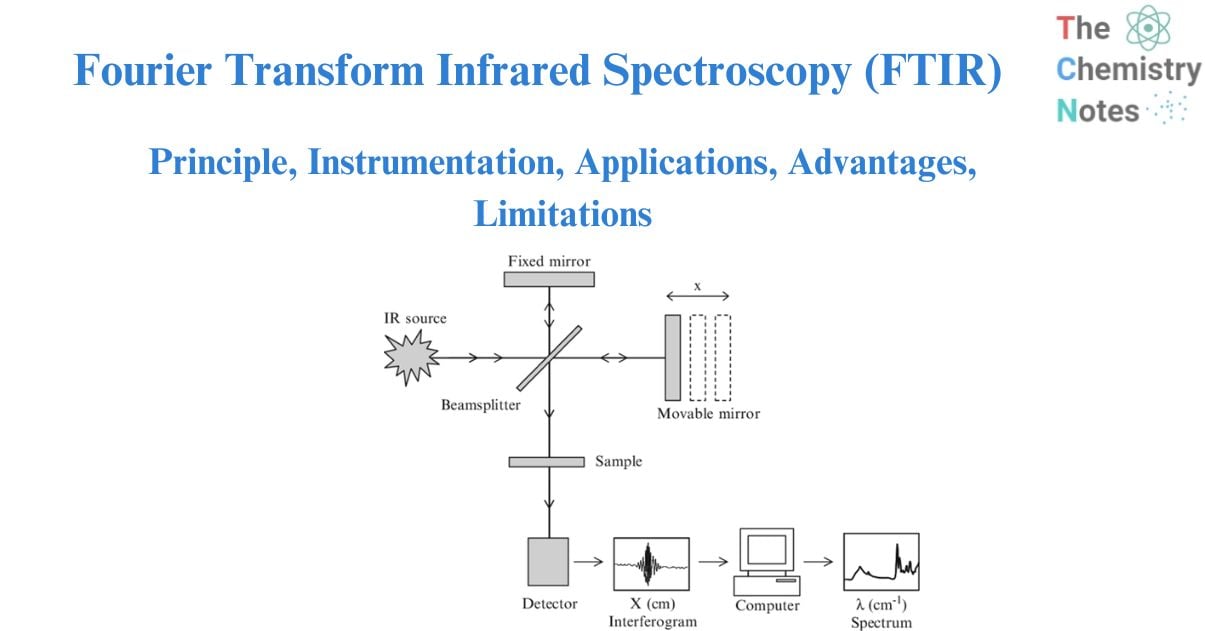
Fourier Transform Infrared (FTIR) spectroscopy is the preferred method of infrared spectroscopy. IR radiation is transmitted through a sample in infrared spectroscopy. The sample absorbs some of the infrared energy, and some of it is transferred (passed through). The resulting spectrum gives the sample a molecular fingerprint by displaying the molecule’s absorption and transmission. No two unique molecule configurations produce the same infrared spectrum, just like fingerprints. As a result, infrared spectroscopy is useful for a variety of analyses.
FTIR (Fourier Transform Infrared Spectrometer) spectrometers are commonly used in organic synthesis, polymer science, petrochemical engineering, the pharmaceutical sector, and food analysis. Furthermore, as FTIR spectrometers can be hyphenated to chromatography, they can be used to explore the mechanism of chemical reactions and the detection of unstable compounds.
Interesting Science Videos
Why FTIR?
For almost seventy years, infrared spectroscopy has been an effective technique for materials analysis in the laboratory. An infrared spectrum is a sample’s fingerprint, with absorption peaks corresponding to the frequency of vibrations between the bonds of the atoms that make up the material.
Because each material is a unique atom combination, no two compounds create the same infrared spectrum. As a result, infrared spectroscopy can provide a positive identification (qualitative analysis) of any type of material. Furthermore, the magnitude of the peaks in the spectrum indicates the amount of material present. Infrared is a useful tool for quantitative analysis when combined with modern software algorithms.
Fourier Transform Infrared (FTIR) spectrometry is designed to overcome the limitations of dispersive equipment. The lengthy scanning procedure was a significant constraint. It is preferable to measure all IR frequencies at the same time. The approach employed a basic optical device known as an interferometer to generate a signal that contained all infrared frequencies “embedded” inside it. The signal is normally recorded in a few seconds.
Most interferometers use a beamsplitter, which separates the entering infrared beam into two optical beams. The signal that emerges from the interferometer is the consequence of these two beams “interfering” with one another since one beam has a fixed route and the other’s path is variable due to the movement of its mirror. An interferogram is the resulting signal. All frequencies are thus measured concurrently when the interferogram is measured. As a result of the interferometer’s employment, measurements are exceedingly quick.
The measured interferogram signal cannot be analyzed directly because the analyst requires a frequency spectrum for identification. It is necessary to have a method of “decoding” the individual frequencies. This is possible because of a well-known mathematical technique known as the Fourier transformation.
For numerous reasons, Fourier transforms infrared spectroscopy is favored over dispersive or filter methods of infrared spectral analysis:
• It is a non-destructive procedure.
• It provides a precise measurement method that does not require external calibration
• It can increase speed by collecting a scan every second
- It can increase speed by collecting scans every second;
- it can increase sensitivity by combining scans taken at intervals of one second to cancel out random noise.
- • It offers higher optical throughput.
- • It has a simplified mechanical design with only one moving part.
Principle of FTIR
The Michelson interferometer consists of a beam splitter, a moving mirror, and a stationary mirror. The beam splitter divides the light beam into two halves, which are reflected by the moving and fixed mirrors before being recombined by the beam splitter.
As the moving mirror makes reciprocating movements, the optical path difference to the fixed mirror changes, causing the phase difference to shift over time. Interference light is created in the Michelson interferometer by recombining the light beams. An interferogram records the intensity of the interference light, with the optical path difference recorded along the horizontal axis.
Instrumentation of FTIR
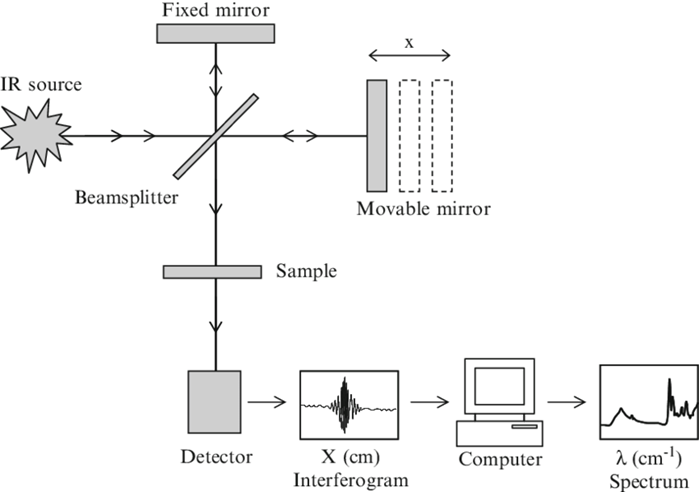
Fig: Instrumentation of FTIR
Image source: https://www.researchgate.net/figure/Schematic-sketch-of-the-essential-features-of-a-Fourier-transform-infrared-FTIR_fig1_225065938
Different parts of FTIR instrumentation include:
The Source: A broadband emitter, such as a mid-IR ceramic source, a far-infrared mercury lamp, or a near-infrared halogen lamp, is used as the light source.
The Interferometer
The interferometer, which consists of a beamsplitter, a stationary mirror, and a moving mirror, is the heart of an FTIR spectrometer. The beamsplitter is a semi-transparent mirror that divides a collimated light beam into two optical channels. Half of the light is transferred to the moving mirror and half is reflected to the stationary mirror. The moving and stationary mirrors reflect the two light beams, which are recombined at the beamsplitter before going through the sample chamber and onto the detector.
The sample
Depending on the type of analysis being performed, the beam enters the sample compartment and is either transmitted through or reflected off the surface of the sample. This is where certain frequencies of energy that are unique to the sample are absorbed.
Detector
FTIR detectors are used to measure and convert the transmitted or reflected light from a sample into an electrical signal. The sensitivity and wavelength range of the data that can be captured is determined by the type and material of the detector.
The detector converts the beam into photons, which are then translated into measurable electric signals that the computer can read. The following are some examples of common detectors:
- Room temperature DLATGS is a regular analysis tool.
- Cooled liquid nitrogen is employed in sensitive applications.
- Si-photodiodes are employed in near-IR and visible infrared applications.
- Silicon far-infrared bolometers
Advantages of FTIR
- It has a higher speed. Because all of the frequencies are detected simultaneously, most FT-IR measurements are completed in seconds rather than minutes. This is also known as Felgett Advantage.
- It has high sensitivity. For a variety of reasons, FT-IR improves sensitivity considerably. The detectors used are much more sensitive, the optical throughput is much higher, resulting in much lower noise levels, and the fast scans allow for the coaddition of several scans to reduce random measurement noise to any desired level.
- It is a very accurate and reproducible method, making it suitable for background subtraction.
- It is an extremely reliable approach for positively identifying almost any material.
- These instruments use a HeNe laser as an internal wavelength calibration standard (the Connes Advantage). These instruments are self-calibrating and never require user calibration.
- It can be used to capture IR data from very small samples.
- It has a high throughput, often known as the Jaquinot advantage. The radiant power reaching the detector is significantly higher than in a dispersive device.
- It has high precision. The laser in an FT-IR spectrometer serves as the instrument’s reference signal and timekeeper. It also moves at the same rate as other components inside its own system. This can provide reliable measurements without being influenced by outside sources such as sunlight or temperature fluctuations.
Applications of FTIR
- FTIR spectroscopy equipment is commonly used to examine industrially manufactured materials in various quality control processes.
- FTIR spectroscopy is a common first step in material analysis. A change in the spectrometer’s absorption band characteristic patterns suggests a change in the material composition or possible contamination.
- It is used to dry polymers, photoresist materials, and polyimides.
- FTIR spectroscopy investigates the interactions between matter and electromagnetic radiation, which appear as a spectrum.
- FTIR spectrum analysis has enabled the diagnosis of various organ diseases as well as the quantification of various biomolecules such as proteins, nucleic acids, and lipids.
- FTIR is a unique approach for characterizing the variation in fuel stability of several biodiesel /antioxidant mixtures.
- In most failure analysis investigations, it is used to determine breakdown, oxidation, and uncured monomers.
- FTIR is also used for :
- high-resolution experiments
- trace analysis in raw materials or final products.
- Reactions on the microsecond time scale.
- Chromatographic and thermogravimetric sample fraction analysis.
- It is used to identify reaction components and conduct kinetic studies on reactions.
- It is used for compound identification by matching the spectra of an unknown substance with a reference spectrum (fingerprinting).
- It is also utilized for functional group identification in unknown compounds. For example, Ketones, Aldehydes, Carboxylic Acids, and so on.
Limitations of FTIR
- The molecule must be active in the infrared range. (When exposed to IR radiation, a minimum of one vibrational motion must change the molecule’s net dipole moment for absorption to be noticed.)
- For the majority of samples, minimal elemental information is provided.
- The material being tested must be transparent in the spectral region of interest.
References
- https://chem.libretexts.org/Bookshelves/Physical_and_Theoretical_Chemistry_Textbook_Maps/Supplemental_Modules_%28Physical_and_Theoretical_Chemistry%29/Spectroscopy/Vibrational_Spectroscopy/Infrared_Spectroscopy/How_an_FTIR_Spectrometer_Operates
- https://jascoinc.com/learning-center/theory/spectroscopy/fundamentals-ftir-spectroscopy/instrumentation/#:~:text=Acquisition%20by%20Interferometer-,Instrumentation%20of%20a%20FTIR%20Spectrometer,source%2C%20interferometer%2C%20and%20detector..
- https://diposit.ub.edu/dspace/bitstream/2445/32136/1/ChT03%20-%20Applications%20of%20FTIR_ed2.pdf.
- https://instrumentalanalysis.community.uaf.edu/wp-content/uploads/sites/279/2013/01/Group2InstrumentInstructionsFT-IR-1.pdf.
- https://epgp.inflibnet.ac.in/epgpdata/uploads/epgp_content/S000831ME/P001676/M030244/ET/1525950185Module-2_Unit5_COM-I.pdf
how i can add your reference in my paper? kindly provide your proper reference for this material please ………