Voltaic or galvanic cells are electrochemical devices that use spontaneous oxidation-reduction reactions to generate electricity. An electrochemical cell called a galvanic cell or voltaic cell, respectively named after the scientists Luigi Galvani and Alessandro Volta. In a typical setup, two different metals are immersed in separate beakers that each contain their own solution of metal ions and are either connected by a salt bridge or separated by a porous membrane. In order to balance the overall equation and highlight the actual chemical transformations, it is frequently convenient to divide the oxidation-reduction reactions into half-reactions when writing the equations.
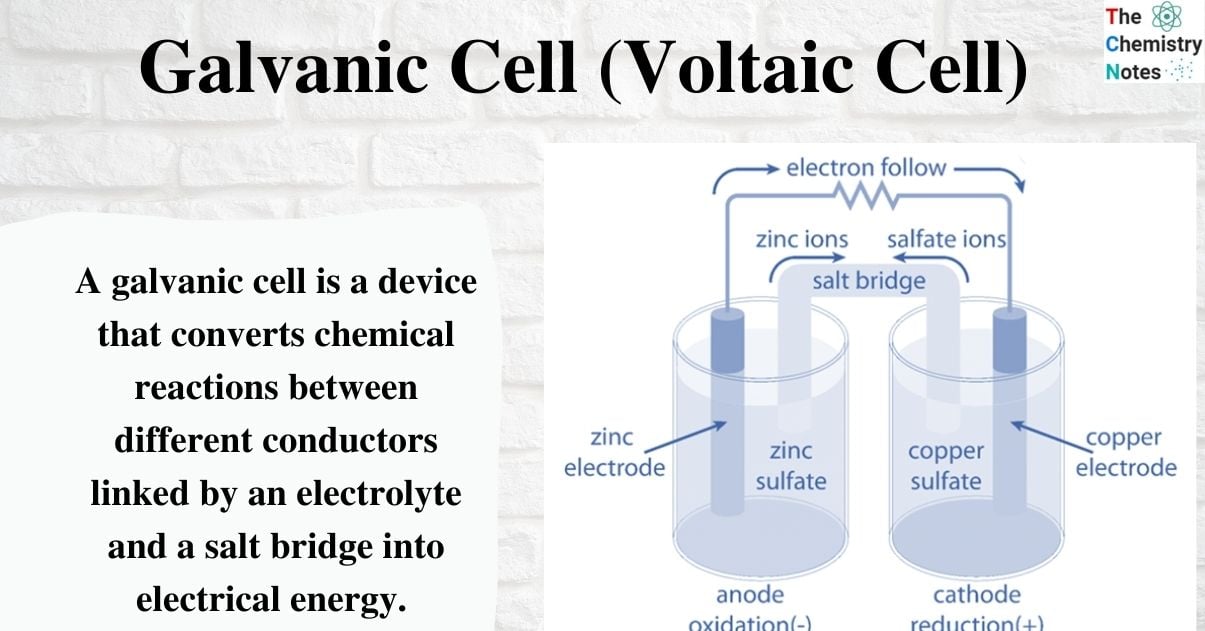
Interesting Science Videos
Galvanic Cell – Definition and Overview
The simplest electrochemical cell, or galvanic cell, transforms chemical energy into electrical energy.
A galvanic cell is a device that converts chemical reactions between different conductors linked by an electrolyte and a salt bridge into electrical energy.
The oxidation-reduction reactions that occur naturally can also power galvanic cells. In essence, a galvanic cell serves as a conduit for the redox reaction’s electrical energy generated by the transfer of electrons.
An electrical circuit, such as one in a television or light bulb, may receive the electrical energy or current. The conductor through which the electrons move is the external circuit. The salt bridge, which balances the charge in each half cell by containing an inert electrolyte, is the internal circuit. Without it, as electrons accumulated in one half of the cell, the cell would become polarized. Two half-cells with an electrode in contact with a solution make up each galvanic cell.
The cathode (+) serves as the electrode for the reduction half-cell while the anode (-) serves as the electrode for the oxidation half-cell.
Anions and cations in the salt bridge move from the cathode to the anode, respectively.
The anode is negative and the cathode is positive.
Constructions of Galvanic Cell
An appropriate reduction electrode and an oxidation electrode are combined to create a galvanic cell, which uses a redox reaction to convert chemical energy into electrical energy. Through a porous diaphragm or a salt bridge, two electrolytic solutions in which electrodes are submerged are connected to one another. A device that makes use of the electrical energy generated in the outer circuit is connected to the two electrodes.
At the oxidation electrode, electrons are liberated, or oxidation takes place. A negative potential is produced by the accumulation of these electrons on the electrode. Due to the reduction process taking place there, a positive potential forms at the reduction electrode.
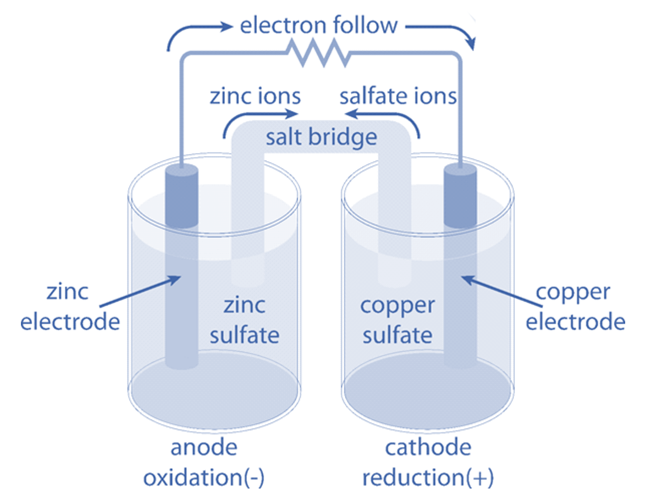
Due to a difference in potential between these two electrodes when they are connected, electrons begin to flow from the oxidation electrode to the reduction electrode in the outer circuit. As a result, an electric current is generated.
The cathode is the electrode where the reduction process takes place, and the anode is the electrode where the oxidation process happens. Anode polarity in a galvanic cell is negative, while cathode polarity is positive.
Galvanic cell components
- Anode: At this electrode, oxidation takes place.
- Cathode: This electrode is where reduction takes place.
- Salt Bridge: Electrolytes needed to complete the circuit in a galvanic cell are contained in the salt bridge.
- Half-cells: Half-cells are divided into compartments for the reduction and oxidation reactions.
- External Circuit: Conducts the flow of electrons between electrodes in an external circuit.
- Load: A component of the circuit uses the flow of electrons to carry out its function.
Working Principle of Galvanic (Voltaic) Cell
The Gibbs energy of the spontaneous redox reaction in the voltaic cell is primarily responsible for the electrical work produced by a galvanic cell.
When an electrode in a galvanic cell is exposed to the electrolyte at the electrode-electrolyte interface, the metal electrode’s atoms tend to produce ions in the electrolyte solution while leaving the electrode’s electrons behind. The metal electrode becomes negatively charged as a result.
Metal ions in the electrolyte solution, however, also have a propensity to deposit on a metal electrode. Consequently, the electrode becomes positively charged.
Under equilibrium conditions, charge separation is seen, and the electrode can be either positively or negatively charged depending on the tendencies of two opposing reactions. As a result, there is a potential difference between the electrode and electrolyte.
Out of two electrodes, the anode is the electrode where oxidation occurs, and the cathode is the electrode where reduction occurs. The cathode has a positive potential concerning the solution, whereas the anode has a negative potential.
Cell Notation
In order to represent a galvanic cell, abbreviated symbolism is frequently used to convey key details about the cell’s make-up and structure. Cell notations or cell schematics are these symbolic representations, and they are written in accordance with the following rules:
- Chemical formulas or element symbols are used to represent the pertinent components of each half-cell.
- When two or more components are present in the same phase, their formulas are separated by commas. All interfaces between component phases are shown as vertical parallel lines.
- According to the convention, the schematic starts with the anode and moves from left to right, identifying the phases and interfaces that are present inside the cell, before ending with the cathode.
- A helpful first step in writing the cell’s schematic is frequently to verbally describe the cell as seen from anode to cathode.
For Example: The galvanic cell in figure below is made up of a solid silver cathode submerged in an aqueous silver(I) nitrate solution, which is connected to a solid copper anode submerged in an aqueous copper(II) nitrate solution by a salt bridge. The cell schematic is produced by translating this statement into symbolism using the aforementioned rules:
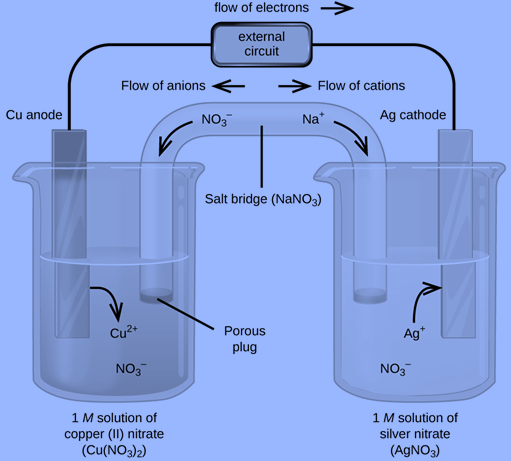

The Electrochemical Series
A galvanic cell’s current flows because one half-cell has a stronger tendency than the other to push electrons into the external circuit. Eo is used to represent a cell’s potential difference which is expressed in volts. The stronger reducing and oxidizing agents are located with the anode and cathode, respectively, in a galvanic cell. By deducting the lower Eo from the higher Eo in the electrochemical series, the Eo value can be calculated.
Electric potential of a Galvanic cell
The electrode potential of a cell can be easily determined by the use of a standard potential table. An oxidation potential table could also be used, but the reduction table is more common. The first step is to identify the two metals reacting in the cell. Then one looks up the Eo (standard electrode potential, in volts) for each of the two half-reactions. The electric potential for the cell is equal to the more positive Eo value minus the more negative Eo value.
For example
The solutions include CuSO4 and ZnSO4. With a salt bridge allowing SO42- ions to freely move between the copper and zinc solutions. When looking up the half-reactions of copper and zinc to calculate the electric potential, one discovers that:
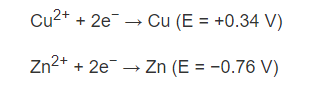
Thus the reaction that is going on is;
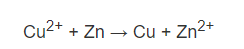
Consequently, the electric potential is +0.34 V -(-0.76 V) = 1.10 V.
If the cell is operated under non-standard conditions, the potentials must be adjusted using the Nernst equation.
Galvanic corrosion
Galvanic corrosion is an electrochemical process in which one metal preferentially corrodes in the presence of another metal when the two metals are dissolved in an electrolyte.
Because different metals have different electrode potentials, a galvanic couple forms when two or more different types of metal come into contact in the presence of an electrolyte. Metallic ions can move from the anode to the cathode through ion migration, which is made possible by the electrolyte. The cathodic metal’s corrosion is slowed down or even stopped as a result, which causes the anodic metal to corrode more quickly than it otherwise would.
The presence of electrolytes and a conducting path between the metals may cause corrosion. If the composition of the electrolyte varies, even a single type of metal may corrode galvanically, creating a concentration cell.
Metals (including alloys) can be arranged in a galvanic series to show the potential that develops between them and a common reference electrode in an electrolyte. Which metal is more likely to corrode more quickly can be determined by comparing two metals’ positions in the series. However, additional variables like water aeration and flow rate can have a significant impact on the process.
Daniell Cell
The best illustration of a galvanic cell that transforms chemical energy into electrical energy is a Daniell cell.
The Daniell cell is made up of two electrodes made of different metals, Zn and Cu, which are each in contact with a solution of their respective ion, zinc sulfate or copper sulfate.
Typically, the Daniell cell is represented as

In a Daniell cell, metal ions move from one half of the cell to the other through the salt bridge while electrons move from the zinc electrode to the copper electrode through an external circuit.
Here, a circuit external to the device allows current to move from a copper electrode to a zinc electrode, or a cathode to an anode.
A voltaic cell can be either reversible or irreversible, whereas the Daniell cell is reversible.
How Daniell Cell is a Galvanic cell?
The best illustration of a galvanic cell is the Daniell cell. A zinc rod dipped in a C1 concentration zinc sulphate (ZnSO4) solution serves as the anode of the Daniell cell. A copper rod is submerged in a solution of copper sulfate (CuSO4) with a C2 concentration to create the cathode.
Both direct contact and a salt bridge create electrical communication between the Zn2+ and Cu2+ ions in the solution.
The copper electrode serves as a cathode, where reduction takes place, and the zinc electrode serves as an anode, where oxidation occurs. The zinc electrode is a source of electrons thus it attracts electrons and forces them into the external circuit. On the other hand, the copper electrode with a low electron density draws the electron from the external circuit. Electrons move from the zinc electrode to the copper electrode as a result.
References
- Atkins, P., “Physical Chemistry”, 6th edition, W.H. Freeman and Company, New York, 1997
- Petrucci, Ralph H.; Herring, F. Geoffrey; Madura, Jeffry D.; Bissonnette, Carey (2017). General chemistry: principles and modern applications (11th ed.). Toronto: Pearson. p. 869. ISBN 978-0-13-293128-1. OCLC 951078429.
- McMurry, John; Fay, Robert C.; Robinson, Jill K. (2015). Chemistry (7th ed.). Boston: Pearson. p. 762. ISBN 978-0-321-94317-0. OCLC 889577526
- https://school.careers360.com/chemistry/galvanic-cell-topic-pge
- https://chem.libretexts.org/Bookshelves/General_Chemistry/Chemistry_1e_(OpenSTAX)/17%3A_Electrochemistry/17.2%3A_Galvanic_Cells
- https://en.wikipedia.org/wiki/Galvanic_cell#Cell_voltage
- https://www.embibe.com/exams/galvanic-cell/
- https://byjus.com/chemistry/daniell-cell/
- https://www.chemeurope.com/en/encyclopedia/Galvanic_cell.html
- https://freevcenotes.com/chemistry/notes/galvanic-cells