Gas Chromatography-Mass Spectrometry (GC-MS) combines two sophisticated methods to identify chemicals. Although GC-MS studies may be used with solid, gaseous, and liquid samples, they are most often limited to volatile and semi-volatile substances. The components of a mixture are separated using gas chromatography, and they are then each separately characterized using mass spectroscopy. An analytical chemist may assess a solution comprising a number of chemicals both qualitatively and quantitatively by combining the two approaches.
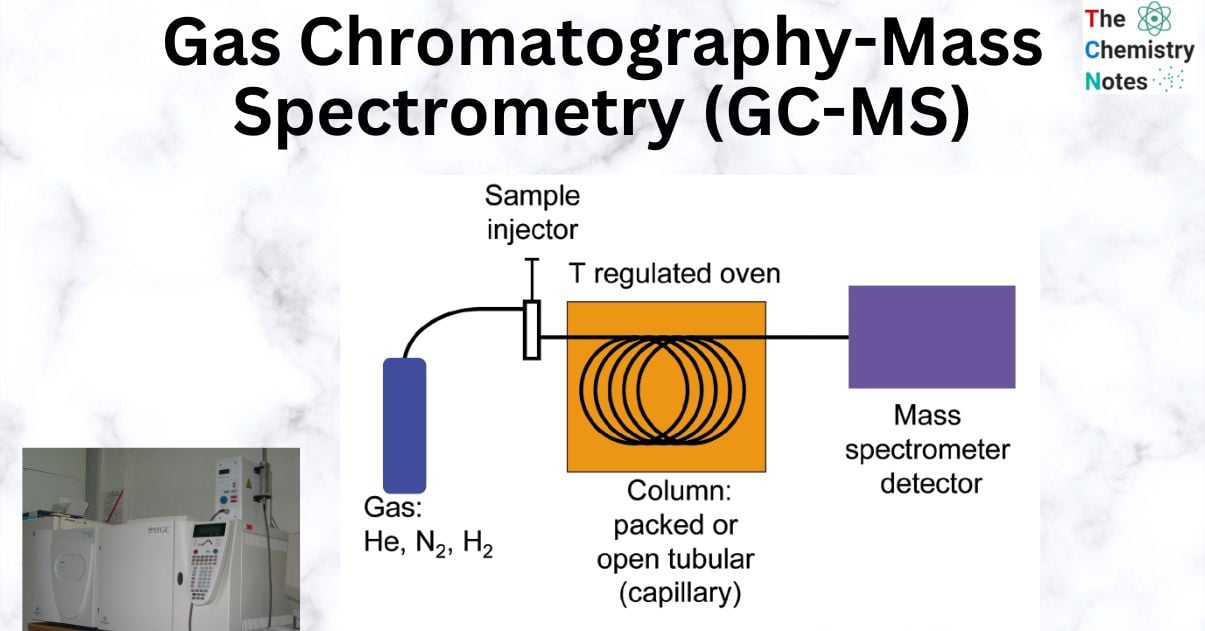
Interesting Science Videos
What is Gas Chromatography-Mass Spectrometry (GC-MS)?
GC-MS is the separation technique of choice for smaller volatile and semi-volatile organic molecules such as hydrocarbons, alcohols, and aromatics, as well as pesticides, steroids, fatty acids, and hormones. Complex mixtures can be separated, analytes can be quantified, unidentified peaks can be identified, and trace contamination levels can be determined when GC is paired with the mass spectrometer (MS) detection power.
An inert gas is used in Gas Chromatography (GC) to carry a sample down a coated glass capillary column after it has been volatilized. The “stationary phase” is adhered to the column’s interior. When compared to a reference, the “retention time”—the amount of time it takes a particular chemical to transit through the column and reach the detector—can be used to identify the substance. Compounds leaving the GC column are fragmented by electron impact during the standard Mass Spectrometry (MS) step of GC/MS. The charged fragments are found, and the resulting spectrum can be utilized to determine the molecule’s identity. Quantitative measurements can be created using reproducible fragmentation patterns.
Principle of Gas chromatography-mass spectrometry (GC-MS)
Gas chromatography-mass spectrometry (GC-MS) is one of the so-called hyphenated analytical techniques. The instruments are connected using a heated transfer line that runs from the end of the column to the entrance to the MS ion source, where chemicals that elute from the column are transformed into ions. Separating, identifying, and quantifying complex chemical mixtures is possible. As a result, it is perfect for analyzing the many relatively low-molecular-weight chemicals that are present in biological and environmental materials. The analytes must be volatilized before being separated using the gas-phase separation technique known as GC. A molecule must be sufficiently volatile and thermally stable in order for Gas Chromatography-Mass Spectrometry to evaluate it.
Geochemical investigations also frequently employ heat extraction and pyrolysis techniques for studying solid samples; while more frequently combined with GC-FID, they can also be paired with GC-MS for characterizing the evolved organic fractions.
History of GC-MS
The first online Gas Chromatography-Mass Spectrometry instruments were released in 1958. The advent of reasonably priced, portable computers in the early 1960s made it possible to greatly reduce both the complexity of this instrument’s use and the time required to examine a sample. The first data-processing units were introduced in 1968, marking the significant advancement in GC-MS. Fused-silica capillary columns were employed for the first time in GC-MS analysis in 1981, and they are still the most popular column today.
By combining GC with MS in 1950, researchers Fred McLafferty and Roland Gohlke from the Dow Chemical Company significantly increased the analytical power of GC. The addition of MS made it possible to analyze each element that left the gas chromatograph independently.
Each component could be identified clearly when the mass spectra and chromatographic peaks were combined. The mass spectrum for each peak helps limit the range of potential component identities for an unknown mixture. If the retention time and mass spectra are identical, known standards can then validate the identifications. Gohlke and McLafferty overcame many obstacles to couple GC with MS. They had to build their own chromatography columns because they weren’t commercially available.
Instrumentation of Gas Chromatography-Mass Spectrometry (GC-MS)
There are several components in a Gas Chromatography-Mass Spectrometry system. To get a decent separation and analysis, it’s important to understand the function of each component. To create a gas flow that moves the chemicals through the column and to the detector, the carrier gas is necessary. To feed high-purity and constant-flow gas to the GC, gas filters and flow regulators are utilized. Good separation and repeatable chromatograms depend on the purity and flow of the carrier gas.
The carrier gas should ideally be inert and free of interference with separation and detection. Some of the often-utilized carrier gases are helium, hydrogen, and nitrogen. The decision mostly relies on the sample, the kind of detector, and the varying features and characteristics of these gases. When selecting carrier gases, one should take into account the necessary separation resolution and efficiency, as well as price and detector compatibility.
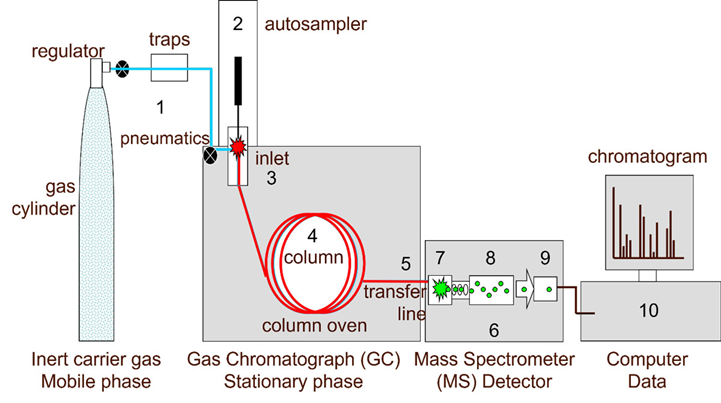
How GC-MS Works?
- The sample is either manually or automatically injected into the GC, and it then enters the carrier gas through the GC intake. If the sample is liquid, the heated GC intake will evaporate it, and the vapor will then be delivered to the analytical column.
- The “analytes,” or components of the sample, are separated based on how differently they partition between the liquid stationary phase (kept inside the column) and the carrier gas, or for more volatile gases, their adsorption by a solid stationary phase. The most typical stationary phase used in GC-MS analysis is a liquid phase contained in a short (10–30 m length) and narrow (0.1-0.25 mm internal diameter) column.
- . The neutral molecules are separated and then elute through a heated transfer line into the mass spectrometer, which is not necessary for GC-MS analysis unless the analytes are isomers.
- The neutral molecules are first ionized inside the mass spectrometer, most frequently by electron ionization (EI). A filament-produced electron is accelerated to 70 electron volts (eV) in electrolysis (EI), where it knocks one electron out of the molecule to create a molecular ion that is a radical cation. The molecule ion produced by this high-energy ionization may be unstable, and any extra energy may be dispersed by fragmentation. A radical or neutral molecule can be lost as a result of bond breakage(s), and chemical rearrangements can also take place. All of this leads to a number of, often a very large number of, ions of varying weights, with the molecule ion being the heaviest and fragment ions having varied lower masses, depending on:
- the chemical composition
- the molecular structure of the analyte
- where bond breakage has occurred
- which component still carries the charge
- The next stage is to separate the ions of various masses, which is accomplished using the mass analyzer depending on their m/z. The mass analyzer’s capacity to differentiate ions with extremely tiny m/z variations is known as mass resolution. High mass resolution (HRMS) instruments can separate nominal masses to four or five decimal places, as opposed to unit mass resolution instruments, which can only separate them to one decimal place.
- The signal is amplified by an electron multiplier (for the majority of low-resolution MS) or a multi-channel plate once the ions have been separated by the mass analyzer based on their m/z when they arrive at the ion detector. Each data point receives a chromatogram and a mass spectrum thanks to the acquisition program on a computer, which records the signal.
Applications of Gas Chromatography-Mass Spectrometry
Gas Chromatography-Mass Spectrometry has a wide range of applications, including the identification of potentially harmful substances in food, quantifying organic pollutants in water, and analyzing petroleum products while refining oil. Forensics, petrochemical, chemical, agricultural, tobacco, pharmaceutical, healthcare, energy, mining, and environmental research, to mention a few, all employ GC-MS to detect unidentified volatile chemicals.
Food and beverage evaluation
In order to guarantee the safety and authenticity of the foods and drinks we consume, GC-MS analysis is essential. Manufacturers and regulatory organizations may benefit from the significant information that GC-MS systems offer on the security of our food supply, including the determination of pesticide residues and the characterization of ingredients.
Analysis of the environment
For monitoring pollutants in air, water, and soil, GC-MS is an effective instrument. It is especially helpful for quantifying polycyclic aromatic hydrocarbons (PAHs), organochlorine insecticides, brominated flame retardants, volatile organic compounds (VOCs), and semi-volatile organic compounds (SVOCs).
Metabolomics
Some of the advanced analytical tools needed for comprehensive metabolomic investigations are provided by metabolomics GC-MS. In order to promote study into primary and secondary metabolites in plants, animals, and microorganisms, it enables researchers to delve deeper into the metabolome and get comprehensive coverage of metabolites.
Analysis of oil and gas
The energy content, CHA, SIMDIS, and H2S/organic sulfur content of natural gas and natural gas condensates may all be determined using GC-MS at various phases of the petroleum and natural gas testing processes. Additionally, oxygenates, aromatics, BTEX chemicals, and PAHs may be found in crude oil using GC-MS analysis in refinery gas analysis (RGA) and detailed hydrocarbon analysis (DHA).
Doping Test
GC-MS is the primary method used in sports anti-doping laboratories to screen urine samples from athletes for illegal performance-enhancing substances such as anabolic steroids.
In medicals
Inborn errors of metabolism (IEM), often known as congenital metabolic disorders, may now be identified by newborn screening tests, particularly those that use gas chromatography-mass spectrometry. Even in urine with low concentrations, chemicals may be identified by GC-MS. These substances are generally absent, although they do show up in people who have metabolic problems. As a result of early diagnosis and the initiation of therapy, this method of IEM diagnosis is increasingly popular and leads to improved outcomes. By doing a urine test at birth based on GC-MS, it is now feasible to screen a baby for over 100 hereditary metabolic diseases.
Limitations and problems Gas Chromatography-Mass Spectrometry
- Leaks into the system and system contamination are the most frequent issues with Gas Chromatography-Mass Spectrometry devices.
- The MS often features a vacuum to lessen background interferences and ion-molecular interactions, extend the lifespan of components, facilitate maintenance, and prevent any electrical discharges because high voltages are employed within the MS. A very strong vacuum is needed to achieve good sensitivity, and while tiny leaks might worsen background levels, larger leaks can hinder the vacuum system. High column flows cause more gas molecules to reach the MS, and because the vacuum system cannot remove them quickly enough to provide a suitable vacuum, sensitivity decreases.
- Therefore, smaller internal diameter columns with lower column fluxes should be employed when converting a technique from GC to GC-MS. The vacuum system of the mass spectrometer determines the maximum flow rate for high sensitivity studies. For the optimum separation effectiveness, this should be understood and taken into account together with the ideal flow rate of the carrier gas.
- In order to maintain good sensitivity, mass spectrometers require routine maintenance, including cleaning the ion source and replacing the oil in the vacuum pump. Instead of moving into the column, where it might harm the stationary phase and contaminate the MS ion source, high molecular weight matrix from the sample should be maintained in the GC input liner, which is readily replaceable, by adjusting the inlet temperature. Column bleed is another cause of ion source contamination. In a mass spectrometer, more stable “-MS” columns should be inserted, and column conditioning shouldn’t be done when the MS is attached.
- Even with little sample preparation, such as when using GC-MS/MS when the matrix cannot be “seen,” GC-related concerns like as activity and sample degradation still arise.
References
- Adlard ER, Handley AJ (2001). Gas chromatographic techniques and applications. London: Sheffield Academic. ISBN 978-0-8493-0521-4.
- Barry EF, Grob RE (2004). Modern practice of gas chromatography. New York: Wiley-Interscience. ISBN 978-0-471-22983-4.
- Eiceman GA (2000). “Gas Chromatography”. In Meyers RA (ed.). Encyclopedia of Analytical Chemistry: Applications, Theory, and Instrumentation. Chichester: Wiley. p. 10627. ISBN 0-471-97670-9.
- Hübschmann HJ (22 April 2015). Handbook of GC-MS : Fundamentals and Applications (3 ed.). John Wiley & Sons, Incorporated. p. 735. ISBN 9783527674336. Retrieved 22 January 2018.
- ^ Hübschmann HJ (22 April 2015). Handbook of GC-MS : Fundamentals and Applications (3 ed.). John Wiley & Sons, Incorporated. p. 731. ISBN 9783527674336. Retrieved 22 January 2018.
- Giannelli PC, Imwinkelried EJ (1999). “Drug Identification: Gas Chromatography.”. Scientific Evidence. Vol. 2. Charlottesville: Lexis Law Publishing. p. 362. ISBN 0-327-04985-5.
- https://www.technologynetworks.com/analysis/articles/gc-ms-principle-instrument-and-analyses-and-gc-msms-362513
- https://www.thermofisher.com/np/en/home/industrial/mass-spectrometry/mass-spectrometry-learning-center/gas-chromatography-mass-spectrometry-gc-ms-information.html
- https://www.acs.org/education/whatischemistry/landmarks/gas-chromatography-mass-spectrometry.html
- https://ceriumlabs.com/what-is-gas-chromatography-mass-spectrometry-gc-ms/