Forces of attraction and repulsion between molecules are known as intermolecular forces. The interactions between molecules of a substance are mediated by these forces. Intermolecular forces are responsible for most of the physical and chemical properties of matter. In general terms, Intermolecular forces are the collective name for the forces that exist between the molecules themselves. Intermolecular forces, which hold the particles that make up solids and liquids together have an effect on a number of the physical characteristics of matter in these two forms.
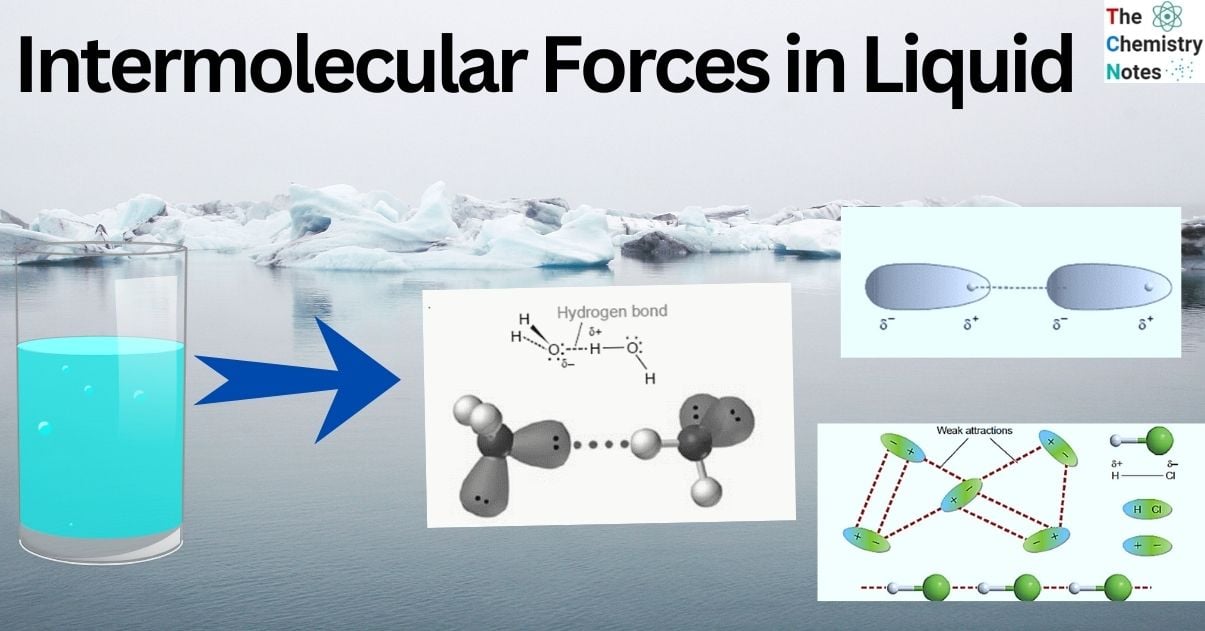
The molecules in liquids are in close contact with each other as compared to gas molecules. The forces of attraction between the molecules are strong enough to hold them together. Most of the physical properties of liquids are actually controlled by the strengths of intermolecular attractive forces. Therefore, before discussing the properties of liquids, we must have a look at the nature of intermolecular forces.
Interesting Science Videos
Intermolecular forces in liquids
An intermolecular force is an attractive force that develops between the protons or positive parts of one molecule and the electrons or negative parts of another molecule. This force affects a substance’s many physical and chemical properties. The strength of a substance’s intermolecular forces directly relates to the boiling point of that substance; the stronger the intermolecular forces, the higher the boiling point. We can compare the intermolecular forces between different substances by comparing their boiling points. This is due to the fact that these intermolecular interactions are disrupted by the heat the substance absorbs at its boiling point, turning the liquid into vapor.
Van der Waals forces and hydrogen bonds are examples of intermolecular forces that are electrostatic in nature. Intermolecular interactions, which keep molecules in liquids attached to one another, are weaker than intramolecular interactions, which keep atoms in molecules and polyatomic ions connected. Changes in intermolecular interactions, but not intramolecular interactions, are what cause transitions between the solid and liquid or the liquid and gas phases.
The three major types of intermolecular interactions:
- Dipole-dipole attractions
- London dispersion forces (often referred van der Waals forces)
- Hydrogen bonding
The relative strength of different intermolecular forces are:
Covalent Bonds | Hydrogen bonding | Dipole-Dipole attractions | London forces |
404 Kcal | 12-16 kcal | 2-0.5 kcal | Less than 1 Kcal |
Dipole-Dipole Attractions
The electrostatic interactions between the positive and negative ends of molecules with permanent dipole moments give rise to dipole-dipole interactions, whose strength is proportional to the magnitude of the dipole moment and to 1/r6, where r is the distance between the dipoles.
Due to differences in the electronegativities of atoms, polar molecules have permanent dipoles when they have covalent bond between the atoms. Thus, polar molecules exhibit dipole-dipole attraction to one another. For this, polar bonds and an asymmetrical molecule are necessary. The positive and negative charges in these molecules are permanently segregated. The image shows that the H and HCl have a tiny persistent positive charge. There is a tiny persistent negative charge at the Cl end of HCl. One molecule’s H atom is drawn to its neighbor’s Cl atom. Compared to a covalent bond, the intermolecular force is minimal, yet this dipole-dipole interaction is one of the stronger intermolecular attractions.
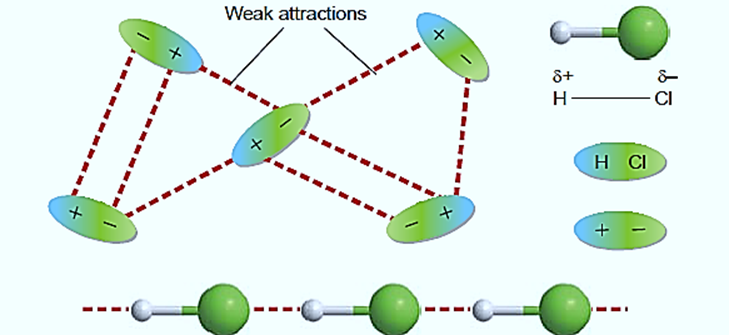
London Dispersion Forces
The short-lived changes in the electron charge distribution that occur in the development of instantaneous dipole moments in polar or nonpolar molecules lead to the temporary formation of an induced dipole in nearby molecules, which is the source of London dispersion forces. Similar to interactions between dipoles, their energy decays as 1/r6. Because their outer electrons are less firmly bonded and consequently more susceptible to perturbation, larger atoms tend to be more polarizable than smaller ones.
Nonpolar molecules exhibit London dispersion forces. These forces are the result of transient charge imbalances. The electrons in a molecule or an ion move randomly within the structure, which accounts for the transient charges. Electrons from the neighboring atom are drawn to the nucleus of one atom. The electrons in one particle repel the electrons in its neighbor at the same time, resulting in a temporary charge imbalance.
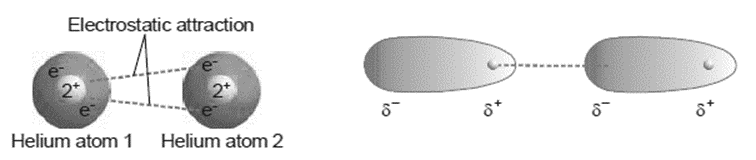
These temporary charges in one molecule or atom attract opposite charges in nearby molecules or atoms. A local slight positive charge δ+ in one molecule will be attracted to a temporary slight negative charge δ– in a neighboring molecule.
London forces in Hydrocarbons and Organic molecules
One nonpolar organic molecule is drawn to its neighbors by the transient separations of charge that result in the London force attractions. The likelihood of these interactions rises as molecular size and surface area increase. The likelihood of “induced” charge separation rises with surface area. The surface area of the molecules is greater if they are straight as opposed to folded into a sphere. Because of the greater attraction, linear molecules have higher melting and boiling points.
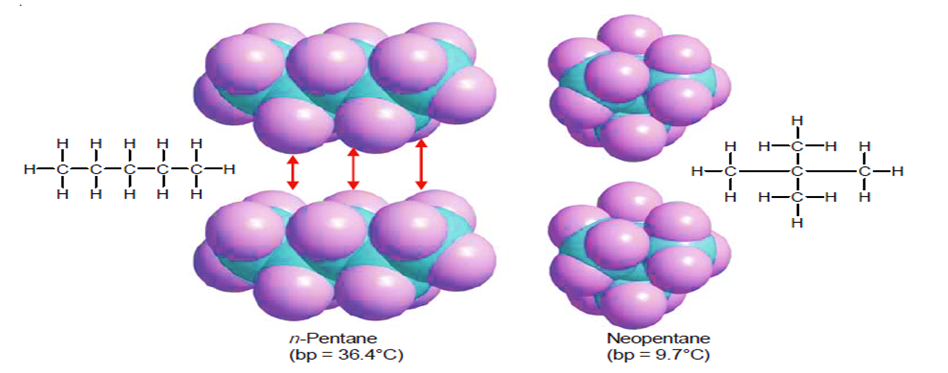
Hydrogen Bonding
Hydrogen bonding is a unique type of intermolecular attraction. There are two requirements:
- Covalent bond between H atom and either an F, O, or N atom. These three elements are the most electronegative ones.
- Interaction between the H atom and a lone pair of electrons on a neighboring element like F, O, or N in this type of polar bond.
Between molecules that have hydrogen bound to a strongly electronegative atom, such as O, N, or F, hydrogen bonds are very potent dipole-dipole interactions. As a result, a partially negatively charged O, N, or F atom on a nearby molecule might interact strongly with a partially positively charged H atom on the resulting molecule. Water has an extraordinarily high boiling point due to the strong O–H hydrogen bonds between its molecules, and ice has an open, cage-like structure that is less dense than liquid water.
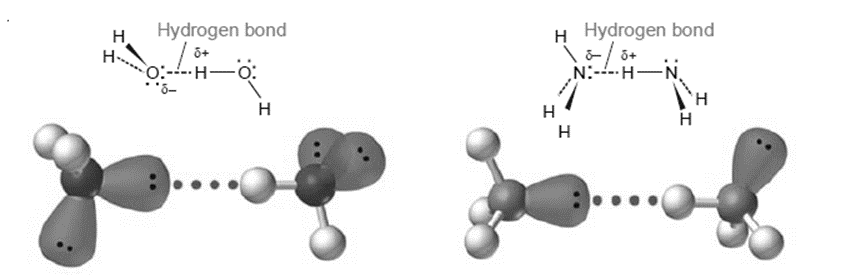
Water typically boils at a temperature of 100oC. When compared to the predicted value, the observed boiling point is high. The expected boiling point is quite low based on the trend of H2Te, H2Se, H2S, and H2O boiling points. The anticipated boiling point would be lower than -62oC if the trend is maintained. Hydrogen bonds between water molecules cause the “anomalous” boiling point for water.
The expansion of water upon freezing is caused by hydrogen bonding. The two lone pairs and two single bonds radiating out from the oxygen are arranged in a tetrahedral pattern in the solid state of the water molecules. Hydrogen bonds can be used to attract the “O” atom’s lone pairs to neighboring water molecules. The outcome is a cage-like structure.
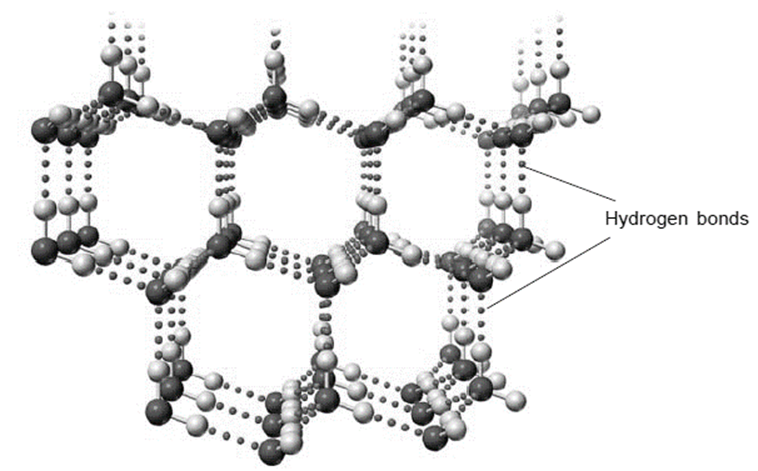
Why do strong intermolecular forces produce anomalously high boiling points and other unusual properties, such as high enthalpies of vaporization and high melting points?
The very polar character of the bonds between hydrogen and extremely electronegative atoms like O, N, and F offers the answer to this query. Due to the significant difference in electronegativity, hydrogen has a significant partial positive charge, whereas the O, N, or F atom has a significant partial negative charge. Since the bond dipoles of H-O, H-N, and H-F bonds are so large, they can interact strongly with one another. These dipoles can also go closer to one another than most other dipoles because hydrogen atoms are so tiny.
Strong dipole-dipole interactions known as hydrogen bonds are produced when massive bond dipoles combine with close dipole-dipole spacing. A tetrahedral structure maximizes the amount of hydrogen bonds that can form since each water molecule has two hydrogen atoms and two lone pairs. Each oxygen atom in the structure of ice is surrounded by a bent tetrahedron of hydrogen atoms that act as bridges to the oxygen atoms of neighboring water molecules. However, the two oxygen atoms that the bridge hydrogen atoms connect are not equally spaced from one another. Instead, each hydrogen atom is 174 and 101 pm from each oxygen, respectively.
In contrast, each oxygen atom forms two covalent connections with H atoms at the shorter distance and two hydrogen bonds with H atoms at the longer distance, which are formed by nearby water molecules. Ice has an open, cage-like structure as a result, which makes the solid somewhat less dense than the liquid. This is why ice floats over water as opposed to sinking.
In order to create an open, cage-like structure, each water molecule takes two hydrogen bonds from two other water molecules and gives two hydrogen atoms to make hydrogen bonds with two additional water molecules. Liquid water has a structure that is very similar to that of solid water, but because of the rapid molecular motion in the liquid, hydrogen bonds are constantly being made and destroyed.
Despite having typical dissociation energies of approximately 15–25 kJ/mol, hydrogen bonds are substantially weaker than covalent bonds and have a considerable impact on a compound’s physical characteristics. Only two hydrogen bonds can be formed at once by substances like HF, on average, and by pure liquid NH3. As a result, although having molecular weights that are comparable to those of water, these substances have boiling temperatures that are substantially lower than those of water, which establish four hydrogen bonds at once.
Watch the Video for better visualization of the concept of intermolecular forces in liquid
References
- Arun Bahl, B. S. Bahl & G. D. Tuli, Essentials of Physical Chemistry, S. Chand and Company Ltd., New Delhi, 2012
- Lange’s Handbook of Chemistry (1967) 10th ed. pp 1661–1665 ISBN 0-07-016190-9 (11th ed.)
- https://chem.libretexts.org/Bookshelves/General_Chemistry/Map%3A_Principles_of_Modern_Chemistry_(Oxtoby_et_al.)/Unit_3%3A_The_States_of_Matter/10%3A_Solids_Liquids_and_Phase_Transitions/10.3%3A_Intermolecular_Forces_in_Liquids#:~:text=Intermolecular%20forces%20are%20electrostatic%20in,within%20molecules%20and%20polyatomic%20ions.
- Petrucci, Ralph H., et al. General Chemistry: Principles and Modern Applications. Upper Saddle River, NJ: Prentice Hall, 2007.
- Halliday, David; Resnick, Robert; Krane, Kenneth S. (2010-04-20). Physics, Volume 2. John Wiley & Sons. p. 342.