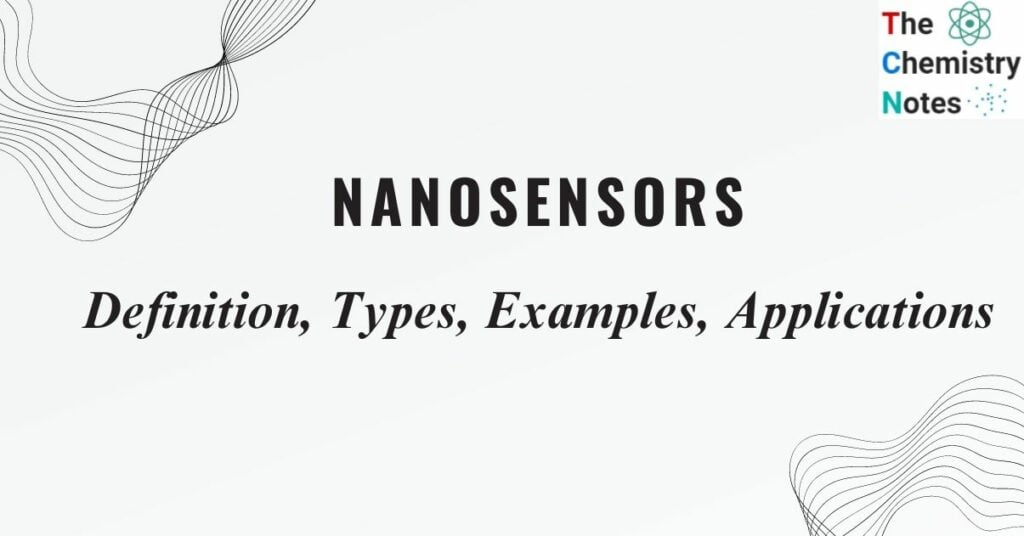
Nanosensors are devices with a characteristic dimension of just a few nanometers, they function similarly to regular sensors in that they detect minute quantities and transform them into signals that must be analyzed.
Materials with nanoscale sizes have lately emerged as exciting possibilities for biological sensing applications. They provide unique insights into real-time changes in critical physiological parameters due to their particular size-dependent physicochemical features.
Nanosensors are employed in pathogen detection, manufacturing, and transportation systems, as well as in medicine, pollution control, and pathogen detection. By detecting physical characteristics like volume, motion, concentration, speed, gravity, magnetic force, pressure, temperature, and electricity, this technology pinpoints the individual cells at the molecular level to give medications and track the growth of particular body parts.
Interesting Science Videos
How do nanosensors work?
Analyte, sensor, transducer, detector, and the feedback line from the detector to the sensor block (if necessary) are the fundamental parts of nanosensors. These sensors can measure single molecule levels and work by maintaining an electrical change in the sensor material.
Typically, they function by monitoring electrical alterations in the sensor materials. The analyte diffuses from the solution to the sensor’s surface and reacts precisely and effectively, changing the surface’s physicochemical properties, which then causes a change in the transducer’s optical or electronic properties. This change is then converted into an electrical signal that is detected.
They are based on nanoparticles and perceive signals such as force, electrochemistry, or biological molecules. they often operate at the nanoscale. Targeting ligands gives their specificity. The nanoparticles are immediately coupled to these ligands. The sensitivity of the system is provided by the nanoparticles, which also transform signals from one form to another or serve as detectors for created signals. Depending on the functionality of the ligand, it may attract a specific marker of interest (analyte).
Bulk nanostructured materials-based nanosensors
While several nanoparticle characteristics can be applied to nanosensors, their catalytic behavior is one of the most crucial in terms of electrochemical sensing devices. For example, platinum nanoparticles supported on noble metals like gold or porous carbon are said to be important in the construction of gas diffusion electrodes.
Nanoparticles are also well suited for immobilizing chemicals, polymers, or biomaterial coatings that enable the creation of composite materials with adjustable surface properties due to their high surface area. For instance, creating new electrochemical sensors with specialized specificities could result from altering metal nanoparticles with prefabricated receptor units and putting them on surfaces. By properly functionalizing nanoparticles, simple and highly selective electroanalytical methods can also be accomplished.
Metal-organic framework-based (MOF) nanosensors
Metal-organic frameworks (MOFs) are organic-inorganic hybrid crystalline porous materials made of an organized array of positively charged metal ions encircled by organic “linker” molecules. The metal ions act as nodes, connecting the linkers’ arms to create a repeating, cage-like structure. MOFs have an extremely large interior surface area due to their hollow structure, making them suitable materials for gas sensing.
Fabrication of nanosensors
Top-down techniques
It is sometimes referred to as sculpting. In this process, basic material is dissolved to obtain the desired shape. This procedure is carried out mechanically using UV light, electron beams, and X-rays or by utilizing acids. In the production of computer chips, this method is used. This technique can be used for lithographic patternings, such as nanoscale printing, molding, and stamping.
Bottom-up techniques
Nanostructures are built using this method from smaller beginning materials such as atoms, molecules, or clusters. These atoms or molecules coalesce into nanometer-sized particles as a consequence of various interatomic or intermolecular forces, van der Waals forces, electrostatic forces, and other short-range forces.
Types of nanosensors
Optical nanosensor
These kinds of sensors are employed for analytical reasons, for monitoring and identifying biological or chemical processes, and to convert the data into signals for significant information. E.g., Proximity Nanosensors
Biological nanosensors
These are employed in bio-molecular processes such as DNA, antigen or antibody contacts, and enzymatic or cellular processes. Eg., Antibody Or antigen interaction nanosensor
Chemical nanosensors
These are used to identify or quantify the amount of a chemical compound. E.g., Molecular concentration nanosensors.
Physical nanosensors
The characteristics of a material, such as force, pressure, displacement, temperature, pressure, and mass, are monitored and measured using these nanosensors. E.g., Pressure type nanosensors, Mass-type nanosensors
Some examples of nanosensors
Some examples of nanosensors are:
- fluorescent nanosensors made of DNA, peptides,
- quantum dots, or carbon nanotubes.
- Nanosensors based on plasmon coupling
- Nanosensors based on magnetic resonance imaging
- Nanosensors based on photoacoustics
Carbon nanotubes
The cylindrically shaped allotrope of carbon is known as a carbon nanotube (CNT). As a result of their use in electronics, optics, and other fields of material science, they are among the most extensively investigated nanostructures. Sensors made of carbon nanotubes have been widely utilized to detect a variety of substances, such as gas molecules and proteins.
Nanowire sensor
They can be used to make ultrasensitive nanosensors for sensing applications because of their distinctive electrical and optical properties. Sensors based on nanowires of various materials have been reported for biosensors, molecule detection, nano-connectors, and gas sensors.
Polymeric nanofiber-based sensors
Polymeric nanofibres have superior mechanical properties, a high surface-to-volume ratio, and flexibility in surface functionalities in comparison to other materials and microfibers. Due to their qualities, they make an excellent choice for a wide range of sensing applications and sturdy structural materials. In comparison to monolithic materials, nanocomposite materials may have superior mechanical strength and structural characteristics.
Applications of nanosensors
- They are used to track metabolic activity and plant signaling in order to comprehend plant biology.
- They are used to investigate brain neurotransmitters in order to understand neurophysiology.
- They are used to gather real-time readings of soil parameters for agricultural use, such as pH, moisture, nutrients, and residual pesticides.
- Graphene and its derivatives are widely used as electrical nanosensing materials. Dopamine, a prevalent neurotransmitter that is utilized as a marker for various disorders, most notably Parkinson’s disease, was detected using graphene oxide.
- Aflatoxin M1 in milk has been detected using an immunochromatographic strip approach based on gold nanoparticles.
- They are crucial to provide real-time monitoring of field and crop conditions, pest attacks, environmental stressors, and plant diseases.
- Although carbon nanotubes (CNTs) are strong, inert structures, their electrical properties are incredibly sensitive to the effects of charge transfer and chemical doping by various molecules, which is because the majority of sensors based on CNTs are field effect transistors (FETs).
- Additionally, chemo-resistive sensors for the detection of illnesses have been constructed using nanowires and nanofibers. They have been used to increase gas sensor responses in exhaled breath analysis for the detection of volatile organic compounds, which serve as biomarkers for several diseases. For instance, acetone, hydrogen sulfide, ammonia, and toluene can be used as biomarkers for assessing diabetes, halitosis, kidney dysfunction, and lung cancer, respectively.
References
- https://www.sciencedirect.com/topics/engineering/nanosensors
- https://www.biomedcentral.com/collections/nanosensors.
- https://link.springer.com/article/10.1007/s10311-017-0616-4
- https://www.ncbi.nlm.nih.gov/pmc/articles/PMC6958676/
- https://pubs.rsc.org/en/content/articlelanding/2019/ra/c8ra10144b