Nanotoxicology is concerned with determining the negative consequences of nanoparticles on human health and the environment. Nanotoxicology seeks to define and identify the hazards of manufactured nanomaterials and necessitates a multidisciplinary team approach that includes toxicology, biology, chemistry, physics, material science, geology, exposure assessment, pharmacokinetics, and medicine.
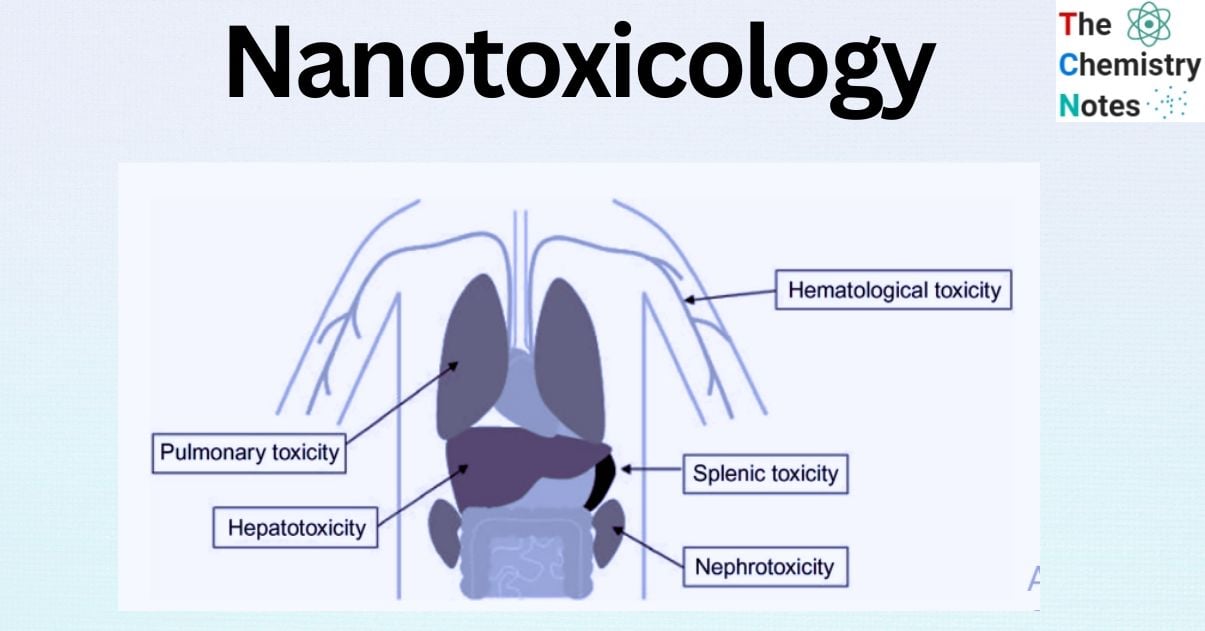
Interesting Science Videos
What is Nanotoxicology?
The study of the negative consequences of created nanomaterials or nanoparticles on living things is the focus of the branch of nanoscience known as nanotoxicology.
The growing use of artificial nanoparticles for biomedical purposes has prompted considerable concerns regarding their safety in humans. Because of their small size and unique features, nanoparticles (NPs) are frequently exploited as nanomedicine and drug carriers. However, their size, morphology, surface functional groups, and dose-dependent features may also play a role in their toxicity to normal, healthy human cells, tissues, and organs.
Nanotoxicology is defined as the study of the nature and mechanism of toxic effects of nanoscale materials/particles on live beings and other biological systems. It also deals with the quantitative measurement of the severity and frequency of nano-toxic effects in proportion to organism exposure. In fact, scientists have been studying the health impacts of exposure to airborne nanoparticles for years and have discovered some unexpectedly negative health effects of nanosized particles in vivo.
For example, epidemiological studies have discovered links between disease incidence and mortality and the amounts and sizes of airborne particles in the environment. The abundance of nanoparticles may cause an increase in mortality. Recently, the biological/toxicological consequences of produced nanomaterials and nanoparticles have been studied.
Mechanism of Nanotoxicology
Nanomaterials in the body can produce toxicity through many molecular pathways. Because of their compact size, NPs are quite popular. This property improves the likelihood of NPs entering organisms and cells without being detained by human body defense mechanisms (e.g., skin mucosa, pulmonary epithelium, macrophages, etc.). Apart from the features of each NP, the presence and severity of any hazardous impact is undeniably dependent on the characteristics of cells and organisms, as well as a number of environmental factors.
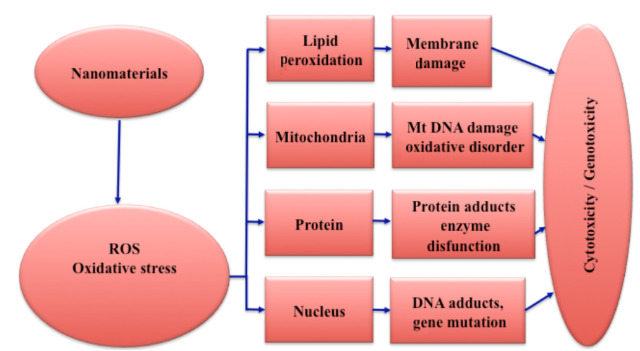
- The most typical impact of NPs-cell contact is the overproduction of Reactive Oxygen Species (ROS), such as Hydroxyl radical (●OH), Singlet oxygen (1O2), Superoxide anion (●O2), Hydrogen peroxide (H2O2), Hypochlorous acid (HOCl), and others.
- It should be mentioned that ROS formation is a physiological process that occurs as a result of normal cell processes or organisms’ exposure to radiation, medicines, or air pollution, among other things. Under normal conditions, humans’ defense mechanisms activate a number of antioxidants to catalyze the oxidative explosion (superoxide dismutase, catalase, glutathione peroxidase, and so on).
- The activation of macrophages and neutrophils once they perceive NPs as a possible threat and mitochondrial disruption are both substantially associated with ROS outburst.
- Cell death in humans and rats, lung and cardiovascular inflammation, and DNA damage are just a few of the negative effects of many NPs linked to ROS generation.
- The primary molecular process is the generation of oxidative stress via free radicals. Oxidative stress may increase the body’s inflammatory response by upregulating reduction-sensitive transcription factors (e.g., NF-B), kinases, and activator protein-1. In excessive quantities, such free radicals can oxidize and hence destroy biological components such as proteins, lipids, and DNA. Because of the delayed elimination and accumulation of free radicals, as well as the presence of a large number of phagocytes, some organs, such as the spleen and liver, are the primary targets of oxidative stress.
- Nanomaterials can also have an impact on other organs with significant blood flow, such as the lung and kidney.
- Other nanotoxicology mechanisms related with nanomaterials may be owing to their high contact with their surroundings. Nanomaterial absorption into the bloodstream can cause thrombosis and hemolysis.
Factor Influencing Nanotoxicology
Size, surface area, shape, aspect ratio, surface coating, crystallinity, dissolution, and agglomeration are NPs features that determine toxicity.
Size and Surface Area
As nanoparticle size lowers, the surface area to volume ratio grows rapidly, increasing biological and chemical reactivities. For example, when the NP size was reduced from 30 to 3 nm, the number of expressed surface molecules increased from 10% to 50%. The interaction between the nanomaterial surface and cellular components causes nanomaterial cytotoxicity. Thus, even though nanoparticles have the same chemical makeup, their cytotoxicity might vary dramatically depending on surface area and particle size. In other words, nanoparticles are more dangerous than larger particles.
In comparison to many microparticles, the body reacts differently to a similar mass made of billions of NPs. The designed nanoparticles have a high surface reactivity as well as a high surface area, which may result in the production of more reactive oxygen species, resulting in cytotoxicity and genotoxicity.
Shape
The shape of nanoparticles is a crucial component in affecting their biological reactivity and toxicity. Nanoparticles are commonly shaped as spheres, cylinders, cubes, sheets, or rods. The form of the nanoparticle has a crucial role in its cellular absorption. The form of gold nanoparticles influences their cytotoxicity and anticancer potential.
For example: Mesoporous silica nanoparticles have demonstrated drug carrier potential in oral medication delivery. Because of their better multiple endocytic processes, internalization rates, and more efficient adhesiveness to the surface of the target cell, needle-shaped nanoparticles are more hazardous than spherical nanoparticles.
Aspect Ratio of Nanomaterials
A nanoparticle aspect ratio is the width to height ratio. A spherical particle has an aspect ratio of one, while a nanotube has an aspect ratio near to zero. The higher the aspect ratio of the NPs, the more poisonous the NPs. In general, aspect-ratio-dependent toxicity is reported in the lung. Asbestosis, mesothelioma, and lung cancer are all linked to nanofibers with a thickness of around 150 nm and a length of 2, 5, and 10 m, respectively. Carbon nanotube samples resulted in substantial protein exudation and granulomas on the peritoneal side of the diaphragm.
Crystallinity of Nanomaterials
The crystalline structure of nanoparticles may influence their toxicity. Polymorphs, which are distinct crystalline forms of the same chemical composition, exhibited varying chemical and physical properties.
Various investigation are done related to the cytotoxicity of 10-hydroxycamptothecin (HCPT) nanoparticle dispersions in both in vivo and in vitro investigations. Three 10-hydroxycamptothecin polymorphic nanoparticle dispersions were created and characterized: pan-cake, prismatic, and needle morphologies. The cytotoxicity data revealed that the cellular toxicities of the various HCPT nanoparticles varied according to size and shape. Despite similar cellular uptakes, needle-shaped HCPT nanoparticles are more powerful in inducing an apoptotic response in cancer cells.
This effect could explain why polymorphs with varied thermodynamic properties, such as lattice energy, are preferred. These results emphasize the importance of accurately characterisation of the polymorphic form (crystalline structure) of nanoparticles for meaningful toxicity evaluation.
Surface Functionalization / Surface Coating
Surface coatings of nanoparticles are used to change their characteristics. A particle’s surface (the “core”) is coated with a layer or layers (the “shell”). The surface coating’s goal may be to alter its stability, wettability, dissolution, or functioning. Because of bioavailability, the surface coating can convert noxious particles to benign particles, while less hazardous particles can become more poisonous. As a result, when compared to non-passivated nanoparticles, there is a reduction in total toxicity during cell internalization.
Agglomeration
Because of their large free surface energy, nanomaterials agglomerate in solution. Nanomaterials are shielded with protective chemicals to minimize aggregation. The toxicity of nanoparticles is also affected by whether or not agglomeration occurs. The aggregation of nanoparticles may be a potential inducer of inflammatory lung diseases in people. The agglomeration-dependent toxicity of nanomaterials is more typically found in carbon nanotubes and oxide nanoparticles. The toxicity of well-dispersed carbon nanotubes is lower than that of agglomerated carbon nanotubes.
Toxic effects of nanoparticles on systems
Because nanoparticles enter the body in three separate routes, experimental studies have shown that they have harmful consequences in various systems.
Circulatory system: In a study of iron oxide nanoparticles administered intravenously to mice it was discovered cardiac oxidative stress and DNA damage. In a research of intravenous injection of Ni nanoparticles in rats, cardiac toxicity-arrhythmia and toxic effects in organs such as the liver, spleen, and lung was demonstrated.
The Digestive System: 100 ppm zirconia oxide nanoparticles cause liver injury in rats. Iron oxide nanoparticles are also harmful to the liver in mice.
Endocrinology: Oral iron oxide nanoparticles cause thyroid hormone abnormalities in rats.
Immune system: Ti02 nanoparticles, induced a significant rise in the number of white blood cells in mice. Furthermore, iron oxide nanoparticles increase the amount of white blood cells, with the liver and spleen being the most immunologically affected organs.
Respiratory system: Metal nanoparticles (Cobalt oxide, nickel oxide, titanium oxide) administered via oropharyngeal aspiration produce toxicity in the lungs.
Urinary system: zinc oxide, iron oxide, and copper nanoparticles are harmful to kidney cells in monkeys, pigs, and cattle. Furthermore, TiO2 nanoparticles given intraperitoneally promote kidney deterioration in rats.
Nervous system: Animal ears and eyes were studied for vision and hearing toxicity, and either minimal toxicity or no toxicity was found in general.
Female reproductive system: Intraperitoneal injection of zinc oxide nanoparticles in mice induced a reduction and loss of seminiferous tubule cells. Furthermore, nickel nanoparticles reduce FSH and LH hormone levels and alter sperm motility in rats.
Future of Nanotoxicology
- Nanotoxicology is a relatively young science that incorporates new advancements in toxicology, such as the use of systems biology techniques to model and forecast perturbations caused by nanoparticles in a biological system.
- Most importantly, nanotoxicology can be viewed as a lesson in interdisciplinarity, as a complete understanding of nano-bio interactions can only be obtained by combining different perspectives found in chemistry, physics, molecular biology, immunology, pharmacology, computational sciences, and so on. As a result, continuous collaboration across scientific disciplines is crucial.
- We must also understand that our students hold the key to the future of nanotoxicology. As a result, preparing the next generation of toxicologists to guarantee that they are well-equipped to cope with more sophisticated compounds is critical.
References
- Toxicity of Nanoparticles in Biomedical Application: Nanotoxicology. PMCID: PMC8376461
- Warheit DB Sayes CM Reed KL Swain KA Health effects related to nanoparticle exposures: environmental, health and safety considerations for assessing hazards and risks. Pharmacology & Therapeutics. 2008;120:35.
- Huang Y.-W., Cambre M., Lee H.-J. The toxicity of nanoparticles depends on multiple molecular and physicochemical mechanisms. International Journal of Molecular Sciences. 2017;18(12):p. 2702. doi: 10.3390/ijms18122702
- Shvedova A. A., Pietroiusti A., Fadeel B., Kagan V. E. Mechanisms of carbon nanotube-induced toxicity: focus on oxidative stress. Toxicology and Applied Pharmacology. 2012;261(2):121–133. doi: 10.1016/j.taap.2012.03.023.
- Oberdörster G., Oberdörster E., Oberdörster J. Nanotoxicology: an emerging discipline evolving from studies of ultrafine particles. Environmental Health Perspectives. 2005;113(7):823–839. doi: 10.1289/ehp.7339.
- The Right Stuff: On the Future of Nanotoxicology Front. Toxicol., Nanotoxicology Volume 1 – 2019 https://doi.org/10.3389/ftox.2019.00001