Nucleophilic substitution reactions are a type of reaction in which one nucleophile attacks a positively charged electrophile to replace another nucleophile.
This reaction takes place by the attack of the nucleophile on the electron-deficient center of the substrate to form the product.
The group on which the substitution occurs is known as the “substrate.” The group with an electron pair displaced from the carbon is known as the leaving group. Generally, a strong nucleophile replaces the weaker nucleophile to give a product.
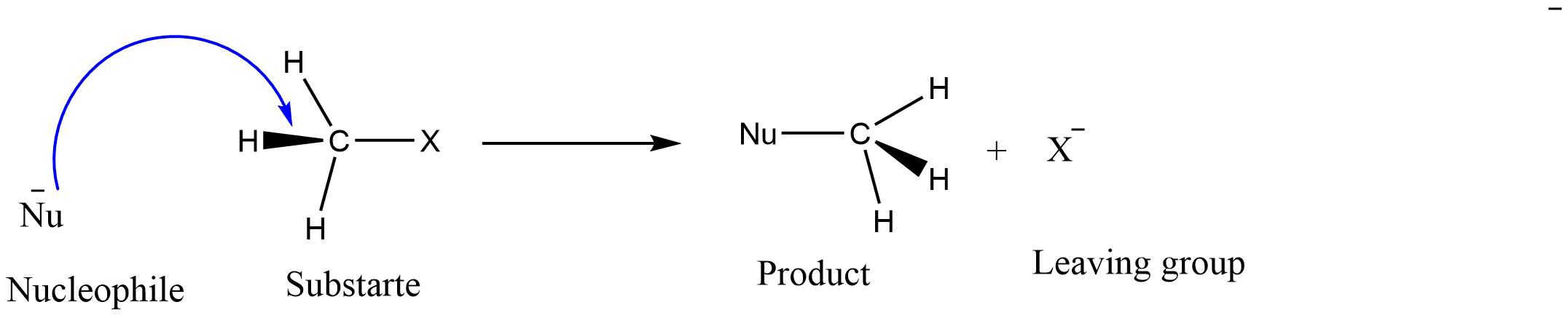
Interesting Science Videos
What is the SN1 Reaction?
It is also known as a unimolecular nucleophilic substitution reaction. Unimolecular nucleophilic substitution consists of a two-step reaction mechanism. In which the formation of carbocation takes place before the nucleophile approaches the substrate. The mechanism is independent of the incoming nucleophile’s nucleophilicity but dependent on the leaving capacity of the leaving group. So, the rate of SN1 reaction depends on the concentration of substrate only.
Commonly the reaction of a tertiary or secondary alkyl halide with secondary or tertiary alcohols under strongly acidic or strongly basic conditions occurs via a SN1 reaction.
Mechanism of SN1 Reaction
The reaction takes place in two steps
a) Step I: Haloalkanes ionize in the solvent to form a carbocation.
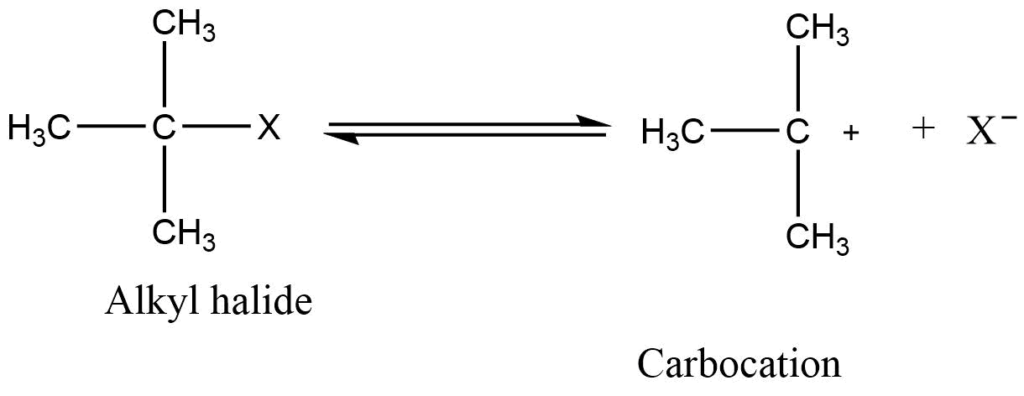
b) Step II: Nucleophile attacks the carbocation to give the product.
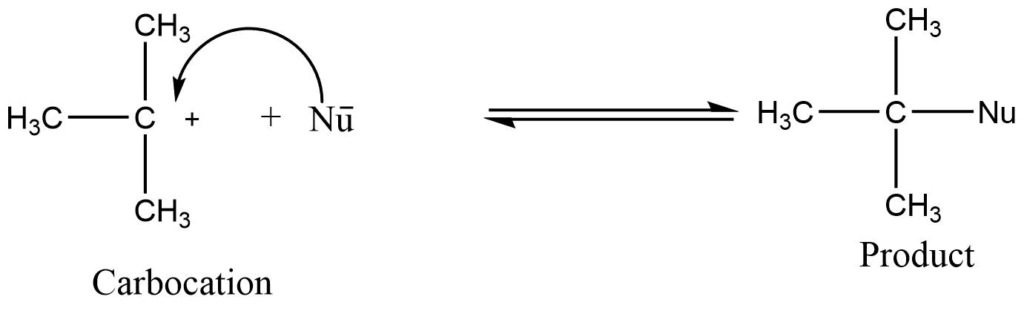
The rate of nucleophilic substitution reaction depends only on the concentration of the substrate and the reaction is of first-order kinetics.
Rate ∝ (substrate)
Example: hydrolysis of tertiary butyl bromide
Features of SN1 Reaction
- Rate of reaction depends on the concentration of the substrate only.
- Rate of reaction depends on the type of substrate and nature of solvent.
- Products having a racemic mixture are formed.
- weak bases generally favor SN1 reaction. They are also good nucleophiles.
- Relative order of reactivity of alkyl halide in an SN1 reaction.
30 > 20 > 10 > methyl halides
6. Rearranged products are possible, carbocation undergoes rearrangement to form a more stable form.
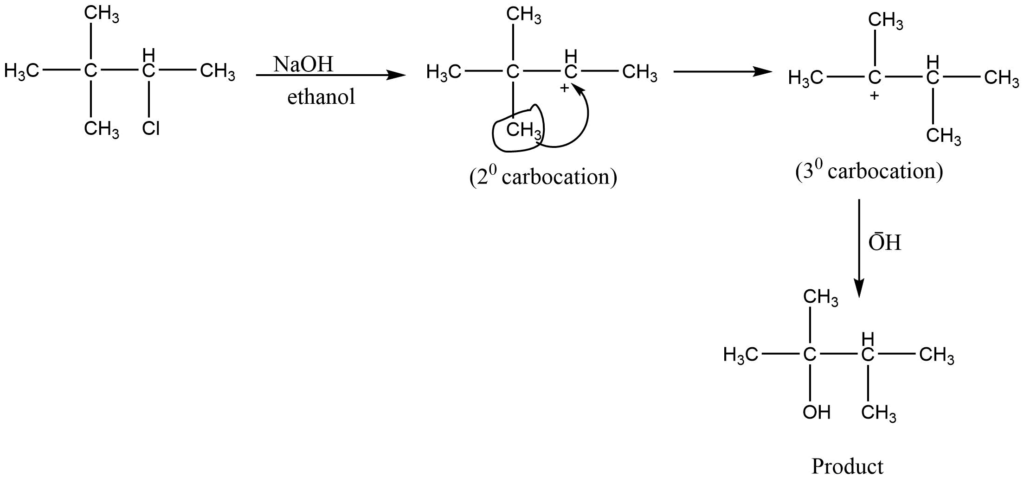
Stereochemistry of reaction
The reaction is proceeded by the formation of an intermediate carbocation in the first step which is sp2 hybridized carbon. This carbocation has a trigonal planar structure. Therefore when it reacts with a nucleophile, it may react from frontside or backside equally.
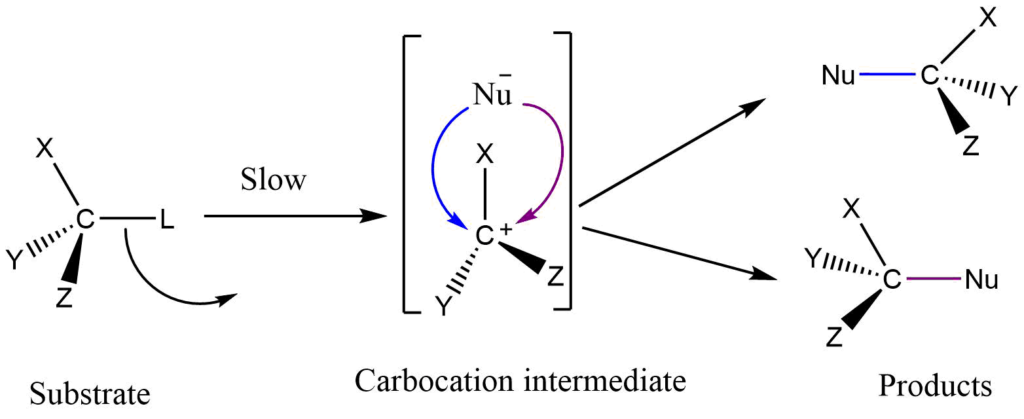
An equal number of molecules with the retention and inversion of configuration in the product are possible. So theoretically it is expected that the product would be racemic.
But experimentally there is always net inversion in the product. This may be due to the attack of nucleophiles before the complete separation of halide ions from the substrate. The separating halide ion shields the frontside attack leading to the product with retention of configuration. This hinders the formation of a product with retention of configuration in comparison to the formation of a product with inversion of configuration obtained from a backside attack. As a result, in the SN1 reaction, inversion of configuration predominates and partial racemization of the product is also possible.
Factors affecting SN1 Reaction
Different factors affect the rate of an SN1 reaction. They are as follows:
Structure of alkyl halides
Alkyl halides can ionize to form stable carbocations. the stability of carbocation follows the order of 3º>2º>1º>methyl. So tertiary alkyl halides are more reactive towards SN1 reaction in comparison to secondary and primary alkyl halides.
Effect of leaving group
Good leaving groups usually prefer SN1 reaction. An SN1 reaction increases with the good leaving group as the leaving group tends to leave the substrate, the bond between the C-Leaving Group breaks faster to form a carbocation. The formation of the carbocation is a rate-determining step, so the faster the formation of carbocation, the faster the nucleophile can enter and the faster the reaction will complete.
Weak bases are a good leaving group as they can hold the charge. Halides are an example of a good leaving group. The leaving ability of the halide group increases as follows:
I– > Br– > Cl– > F–
Effect of solvent
The solvents which facilitate the formation of the carbocation intermediate increase the reaction process. Polar and protic solvents increase the SN1 reaction as the polarity of the solvent aids in the stabilization of ionic intermediates, whereas the protic nature of the solvent helps in the solvation of the leaving group.
Effect of nucleophile
The rate-determining step does not involve the attacking nucleophile, so the strength of the nucleophile does not affect the rate of an SN1 reaction. Usually, the weak nucleophiles favor the SN1 reaction.
Ion pairs in SN1 reaction
The stereochemical evidence of SN1 reaction is quite confusing. If there is a presence of planar carbocation intermediate the nucleophile should attack from either side of the plane resulting in complete racemization.
Though most of the first-order substitutions give complete racemization, in some cases there is a presence of 5 to 20 % inversion and some amount of retention of configuration. This indicates that in many SN1 reactions at least some of the reaction products form via ion pair mechanism rather than the carbocation intermediate.
Reaction process
According to this concept the SN1 reactions proceed by this process
R-X ⇌ R+ X– ⇌ R+ X– ⇌ R+ + X–
R+ X– = tight ion par (intimate ion pair)
R+ X– = solvent separated ion pair
R+ + X– = the dissociated ions
An internal return occurs when intimate ion pair combines to give the original substrate. The product can result from an attack by the nucleophile at any stage.
The attack of the nucleophile at various stages results in different stereochemistry of the product.
- In the tight ions pairs, X– shields the front side attack of the nucleophile. So nucleophiles are more likely to attack from the backside leading to an inversion of the configuration of the product.
- The attack of a nucleophile in solvent separated ion pair can take place with equal facility from either side leading to the racemization of the product.
- In the dissociated ion nucleophile can preferably attack from both side resulting in the racemization of product. As the stability of R+ increases the power of attacking nucleophiles decreases, so the proportion of dissociated ions increases.
In the overall process the degree of racemization increases while the inversion decreases.
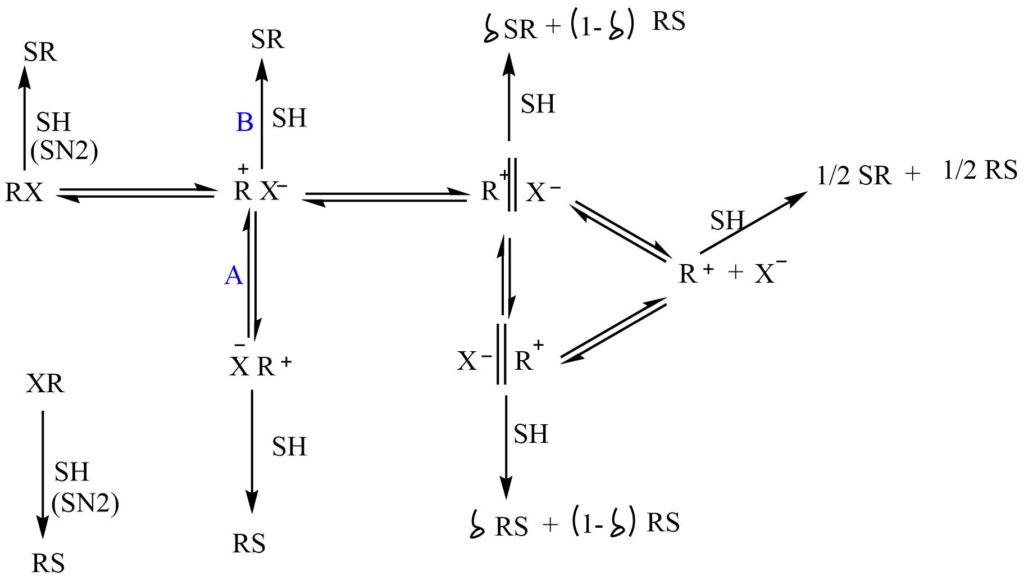
In this reaction process, RS and SR represent the enantiomers and 𝛿 represents some fraction.
Different possibilities of attack of nucleophiles
1. Direct attack by SH on RX occurs through the SN2 process and gives inversion of configuration.
2. In intimate ion pair R+X–, there is a total inversion in configuration if reaction A doesn’t take place. If there is competition between reactions A and B the combination of inversion and racemization takes place.
3. If solvent separated ion pair is formed, SH can attack here. More racemization is expected.
4. In free R+ attack by SH give complete racemization.
So, the ion pair concept predicts that the SN1 reaction can result in either complete racemization or partial inversion of the product.
The evidences of the ion pair mechanism are:
1. The special salt effect: The addition of LiClO4 or LiBr to the acetolysis of certain tosylates resulted in an initial rate acceleration, which was then reduced to normal linear acceleration due to the ordinary salt effect.
2. On solvolysis of 2- octyl brosylate (labeled at the sulfone oxygen with 18O), unreacted brosylate recovered at various stages with 18O significantly but not completely scrambled.
This possibility must be due to the scrambling caused by the ionization of one molecule of ROSO2Ar to R+ and ArSO2–, followed by an attack by ArSO2– ion on another carbocation or possibly on the molecules of ROSO2Ar in the SN1 process.
However, experiments revealed some intermolecular exchange (3-20%) in the solvolysis of the unlabeled substrate in the presence of labeled HOSO2Ar, but not nearly enough to account for the amount of scrambling found in the original experiment.
Also watch this video for more information:
References
- Sykes, P. (1975). A guidebook to mechanism in organic chemistry. New York: Wiley.
- March, J. (1992). Advanced organic chemistry: Reactions, mechanisms, and structure. New York: John Wiley & Sons.
- https://byjus.com/chemistry/sn1-reaction-mechanism/
- https://chem.libretexts.org/Courses/Brevard_College/CHE_202%3A_Organic_Chemistry_II/04%3A_Substitution_and_Elimination_reactions/4.07%3A_Factors_Affecting_the_SN1_Reaction
- https://web.iit.edu/sites/web/files/departments/academic-affairs/academic-resource-center/pdfs/SN1_SN2.pdf
- https://www.masterorganicchemistry.com/2011/09/26/introduction-to-substitution-reactions/
- https://www.britannica.com/science/unimolecular-nucleophilic-substitution-reaction
- https://www.vedantu.com/iit-jee/nucleophilic-substitution-reaction