Nanotechnology is a top research priority in many countries because to its significant potential and economic effect. Nanotechnology is the study, creation, and processing of nanoscale structures and materials in numerous domains of science.
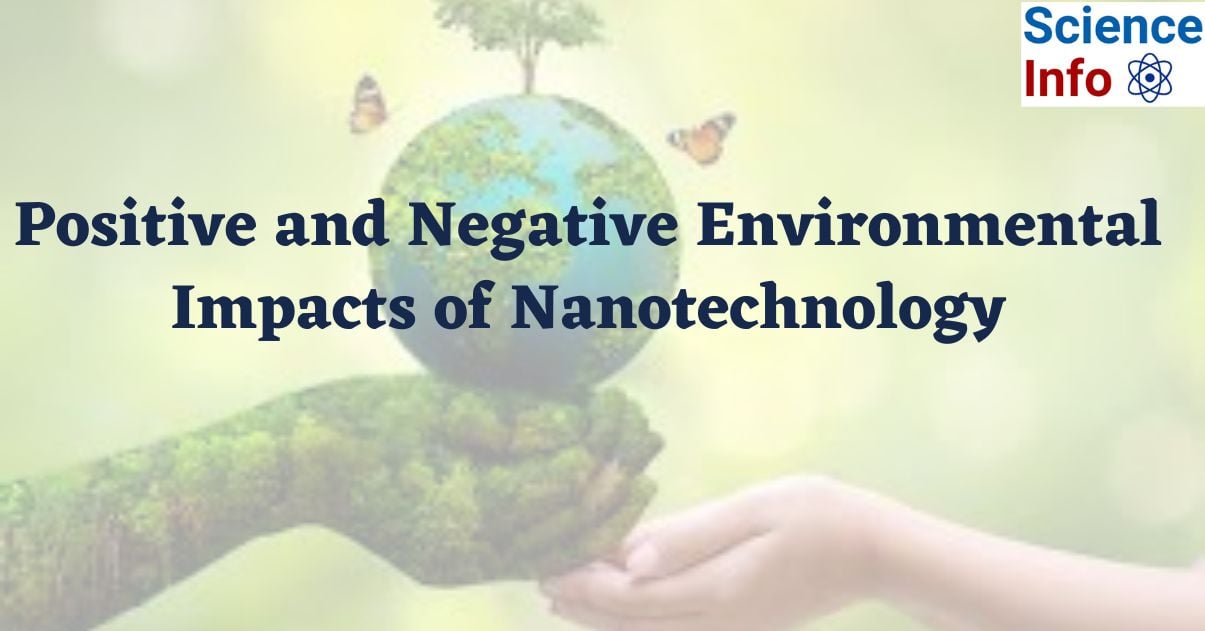
Everything related to nanotechnology, applications, goods, or processes is projected to benefit the environment by conserving energy, water, and raw materials while reducing dangerous greenhouse emissions. As a result, using nanomaterials promises to have long-term effects, environmental benefits, and so on. Nanotechnology has multiple distinguishing characteristics, making it an excellent choice for a wide range of applications, products, and processes. In terms of the environment, nanotechnology offers a new perspective and method to reducing waste and emissions.
Nanotechnology is being extensively examined around the world to determine its potential benefits in a variety of domains, including engineering, biology, chemistry, computing, materials science, and communications. Although they have influenced the evolution of technology, healthcare, industry, and agriculture, resulting in the progressive reorganization of many current technologies, certain nanomaterials may harm both the environment and human health due to their irregular shape, size, and chemical composition. This issue has prompted the globe to pay more attention to the potential environmental impacts of nanotechnology.
Interesting Science Videos
What is Nanotechnology?
Nanotechnology is a discipline of science and engineering that designs, manufactures, and uses structures, devices, and systems by manipulating atoms and molecules at the nanoscale, i.e. with one or more dimensions of 100 nanometres (100 millionths of a millimeter) or less.
There are several instances of structures with one or more nanometer dimensions in nature, and many technologies have used such nanostructures inadvertently for many years, but it has only lately become viable to do so on purpose.
Many nanotechnology applications include new materials with radically different properties and effects than those developed at bigger sizes. This is owing to nanoparticles’ extremely high surface-to-volume ratio as compared to larger particles, as well as effects that emerge at that small size but not at higher scales.
Nanotechnology applications can be extremely useful, with the potential to significantly alter society. Nanotechnology has already been adopted by industrial sectors such as information and communications, but it is also employed in food technology, energy technology, and certain medicinal items and treatments. Nanomaterials may also provide new ways to reduce environmental pollution.
However, these novel materials may also pose new health dangers. Humans have developed protective systems against a wide range of environmental contaminants. Until recently, however, they had never been exposed to manmade nanoparticles or their unique properties. As a result, conventional human defense mechanisms such as the immunological and inflammatory systems may be unable to appropriately respond to these nanoparticles. Moreover, nanoparticles have the potential to spread and endure in the environment, which means they can affect it.
Negative Environmental Impacts of Nanoparticles
Absorption and Distribution
- Nanoparticles (NPs) can enter the environment through various routes, such as respiratory, gastrointestinal, or dermal exposure.
- The properties of living organisms influence how NPs enter the body; for example, nanotubes can easily penetrate the pulmonary epithelium.
- Prokaryotic cells have some protection against NPs due to their cell wall structure, while eukaryotic cells can absorb NPs via phagocytosis or endocytosis.
- Once inside cells, NPs can cause toxicity and harm to different cellular compartments, such as mitochondria, membranes, lysosomes, and the Golgi complex.ROS (Reactive Oxygen Species)
- formation is a significant mechanism of harm caused by NPs.
Toxicity Means: Oxidative Stress
- ROS production and oxidative stress are primary mechanisms of toxicity for NPs, even at low exposure concentrations.
- NPs interact quickly due to their high surface area, leading to ROS generation that damages cellular components like lipids, proteins, and nucleic acids.
- NPs can induce ROS formation in immune system cells, exacerbating oxidative stress.
- Oxidative stress can lead to cell death and DNA damage, including DNA adducts, chain disruption, and mutations.
Bacterial Toxicity
- NPs can affect bacterial cell walls and induce oxidative stress, leading to toxicity.
- Different bacterial species exhibit varying sensitivities to NPs, influenced by factors like cell wall structure and oxidative processes.
- NPs can disrupt bacterial communities and affect nutrient cycling in ecosystems, leading to ecological imbalances.
- Some bacteria may develop resistance to NPs, mitigating their toxic effects at lower concentrations.
- Ag-NPs, known for their antibacterial properties, can impact bacterial growth and metabolism, affecting nutrient cycling in soils and aquatic environments.
Plant Toxicity
- Plants can absorb NPs through various routes, such as roots, leaves, or damaged tissues.
- NP toxicity studies in plants often focus on parameters like seed development, root growth, and nitrogen fixation.
- NPs in soil can accumulate in plants and transfer through the food chain, impacting various trophic levels.
- Different sizes and types of NPs exhibit varying degrees of toxicity to plants, affecting growth, photosynthesis, and nutrient uptake.
- Ag-NPs, TiO2-NPs, and ZnO-NPs are among the NPs studied for their effects on plant health and productivity.
Animal Toxicity
- NPs can impact animal health through various exposure routes, including ingestion, inhalation, and dermal contact.
- Studies in animal models reveal NPs’ potential for inducing genotoxicity, cytotoxicity, and reproductive toxicity.
- Aquatic organisms like fish and invertebrates can experience tissue damage, oxidative stress, and physiological disruptions due to NP exposure.
- NPs can accumulate in vital organs of animals, leading to systemic toxicity and impaired immune function.
- Different species and life stages exhibit varying sensitivities to NPs, influenced by factors like particle size, shape, and chemical composition.
Human Toxicity
- NPs can pose risks to human health through inhalation, dermal contact, or ingestion, with potential adverse effects on the respiratory, cardiovascular, and central nervous systems.
- Limited data on NP exposure in humans necessitates further research to understand potential health effects.
- Occupational and environmental exposures to NPs are of concern, particularly in industries where NPs are manufactured or used.
- Skin contact with NPs, such as those in cosmetics or textiles, raises questions about dermal penetration and systemic effects.
- Oral exposure to NPs through contaminated food or water may lead to systemic distribution and potential toxicity in various organs and tissues.
Although NPs’ potential negative influence on the environment is important, they also have a positive impact that should be carefully evaluated. NPs can have a positive impact on their surroundings through some of their applications. Among the many potential activities that NPs can perform to reduce pollution and improve environmental health, such as promoting energy-efficient production systems or assisting in the process of water and soil remediation, we have focused on those that are highly relevant and interesting in current research.
Positive Impacts of Nanoparticles
Soil and Water Pollution
- NPs can be used to reduce soil and water pollution, including remediation techniques that reduce the use of polluting materials.
- Nanotechnology can reduce the amount of fertilizers, herbicides, insecticides, and growth promoters needed while increasing their action. This reduces the dose required to produce the effect, as well as the associated side effects and pollutant release into the environment, and thus the energy required to carry out further soil treatments.
- Examples include the use of Zn and Al NPs as coating agents to generate a regulated release of nutrients, as well as the use of mesoporous silica to preserve the pesticide’s active components from photodegradation.
- Nanomaterials can be employed as adsorbents, catalysts, membranes, and sensors to absorb and treat contaminants in the atmosphere.
- High surface area nanomaterials improve adsorption capability, making them useful for capturing CO2 via filtration, liquid absorption, and solid adsorption.
- Nanocatalysts help break down and convert pollutants such as CH4 and NOx, leading to cleaner air.
- Nanofilters and membranes can remove pollutants, pathogens, and contaminants from water, including heavy metals, organic compounds, and microbes.
- Nanosensors monitor water quality in real time, detecting pollutants and toxins in distribution networks and bodies of water.
- Nanoremediation technologies, such as in situ remediation with nanomaterials like carbon nanotubes (CNTs) and graphene oxide (GO), provide cost-effective and efficient options for cleaning polluted water sources.
- Soil remediation has emerged as one of the most important applications for nanoparticles in preventing environmental degradation. Zn NP, which has the ability to effectively breakdown dyes and pharmaceuticals, is one type of nanomaterial that can be used in this remediation procedure.
- Iron nanoparticles for eliminating heavy metals or organochlorine chemicals via electronic donation have also been investigated. In this regard, FeO NPs have been shown to be effective in Cr(VI) adsorption, with 98.1% effectiveness.
- Metallic iron has shown to be one of the most interesting materials for decontamination and water treatment, with applications including organochlorine compounds, arsenic, and petroleum derivatives.
Energy Applications
- Nowadays, the growing demand for energy encourages researchers to discover new ways to obtain energy in an environmentally friendly manner. In this way, nanotechnology has become a chance to create clean and renewable energy sources.
- Solar photovoltaic energy derived from solar radiation has emerged as the most intriguing energy source due to its efficiency, adaptability, and ease of application, all of which have minimal influence on natural resources such as water and soil.
- Some of the primary issues with photovoltaic cells include high production costs and low solar energy absorption efficiency, with just 40% converting to electric energy.
- Carbon nanotubes have been investigated as an alternate material for cell structure since they are easily synthesized and can efficiently obtain and store energy. Furthermore, they can endure temperature fluctuations and unexpected shocks.
- TiO2 NPs are also gaining popularity due to their outstanding adsorption and photocatalytic activity, making them a potentially useful material for solar energy collection.
- Carbon nanotube use in windmills, on the other hand, has been shown to have various benefits, including light-weighted blades that can be increased to absorb energy or as base products for antifreeze coatings, which extend the life of windmills in cold areas.
- Geothermal energy extraction is also preferred with the use of some NPs that reduce the depth and temperature required to collect this energy.
Nanotechnology-Based Radioactive Waste Cleanup:
- One potential nanotechnology option for radioactive waste cleanup is to employ titanate nanofibers as absorbents to extract radioactive ions from water.
- Additionally, scientists have discovered that titanate nanotubes (TNTs) and nanofibers have remarkable mechanical qualities that make them appropriate materials for the removal of cesium and iodine ions from water.
Artificial Photosynthesis: Turning Sunlight Into Hydrogen
- Businesses that run on hydrogen want to brag about how green their technology are and how they are saving the environment. Despite its image as a clean fuel, hydrogen comes from sources that are frequently extremely unclean. Although it cannot be extracted from a well, hydrogen can be created via a variety of methods.
- Artificial photosynthesis is a clean, portable energy source that can run on solar energy for as long as the sun. It works by splitting water and producing hydrogen and oxygen.
Other Applications
- Sensors located in various environmental compartments are very effective instruments for detecting toxins, such as pesticides, antibiotics, and microbes. Because of their high surface and reactivity, the use of nanomaterials for this reason improves certain of the sensors’ properties, such as sensibility or precision.
- Although this technology is still in its early phases, several studies suggest that the use of metallic NPs (such as gold, silver, or cobalt NPs) could result in a significant improvement in actual sensors.
- On the other hand, despite the increased availability of clean and renewable energy sources, the majority of the industry continues to utilize fossil fuels, necessitating the reduction of the damage they do by collecting and storing a portion of the CO2 emitted into the atmosphere.
- Nanotechnology has emerged as a distinct approach to this challenge, and numerous solutions have been examined, such as the use of high permeability and selectivity polymeric membranes containing nano-holes or the use of ZIF (Zeloitic Imidazolare Frameworks) NPs.
Vidoe on Environmental Impacts of Nanotechnology
References
- https://builtin.com/hardware/nanotechnology
- MartÃnez G, Merinero M, Pérez-Aranda M, Pérez-Soriano EM, Ortiz T, Begines B, Alcudia A. Environmental Impact of Nanoparticles’ Application as an Emerging Technology: A Review. Materials (Basel). 2020 Dec 31;14(1):166. doi: 10.3390/ma14010166. Erratum in: Materials (Basel). 2021 Mar 31;14(7): PMID: 33396469; PMCID: PMC7795427.
- Bradford, S. A., Shen, C., Kim, H., Letcher, R. J., Rinklebe, J., Ok, Y. S., & Ma, L. (2022). Environmental applications and risks of nanomaterials: An introduction to CREST publications during 2018–2021. Critical Reviews in Environmental Science and Technology, 52(21), 3753–3762. https://doi.org/10.1080/10643389.2021.2020425
- Front. Environ. Sci., 24 May 2018 Sec. Green and Sustainable Chemistry
Volume 6 – 2018 | https://doi.org/10.3389/fenvs.2018.00034 - Mansoor Ahmad Bhat, Kadir Gedik, Eftade O. Gaga, Chapter 23 – Environmental impacts of nanoparticles: pros, cons, and future prospects, Editor(s): Munir Ozturk, Arpita Roy, Rouf Ahmad Bhat, Fazilet Vardar-Sukan, Fernanda Maria Policarpo Tonelli, In Micro and Nano Technologies, Synthesis of Bionanomaterials for Biomedical Applications, Elsevier, 2023,Pages 493-528, ISBN 9780323911955, https://doi.org/10.1016/B978-0-323-91195-5.00002-7.
- https://publikationen.bibliothek.kit.edu/1000046381