Scanning electron microscopy (SEM) is one of the most popular and widely used techniques for the characterization of nanomaterials and nanostructures. With a magnification range of 10 to over 300,000, SEM can properly analyze specimens down to a resolution of a few nanometers. Surface-enhanced microscopy (SEM) can reveal not just surface topography but also chemical composition, crystal orientation, and stress distribution within a material.
Similar to how light microscopes utilize light for imaging, electron microscopes use electrons. SEMs create an image by using electrons that are reflected or knocked off the near-surface region of a sample, as opposed to TEMs, which detect electrons that pass through a very thin specimen. SEMs have better resolution than light microscopes because electrons have a considerably smaller wavelength than light.
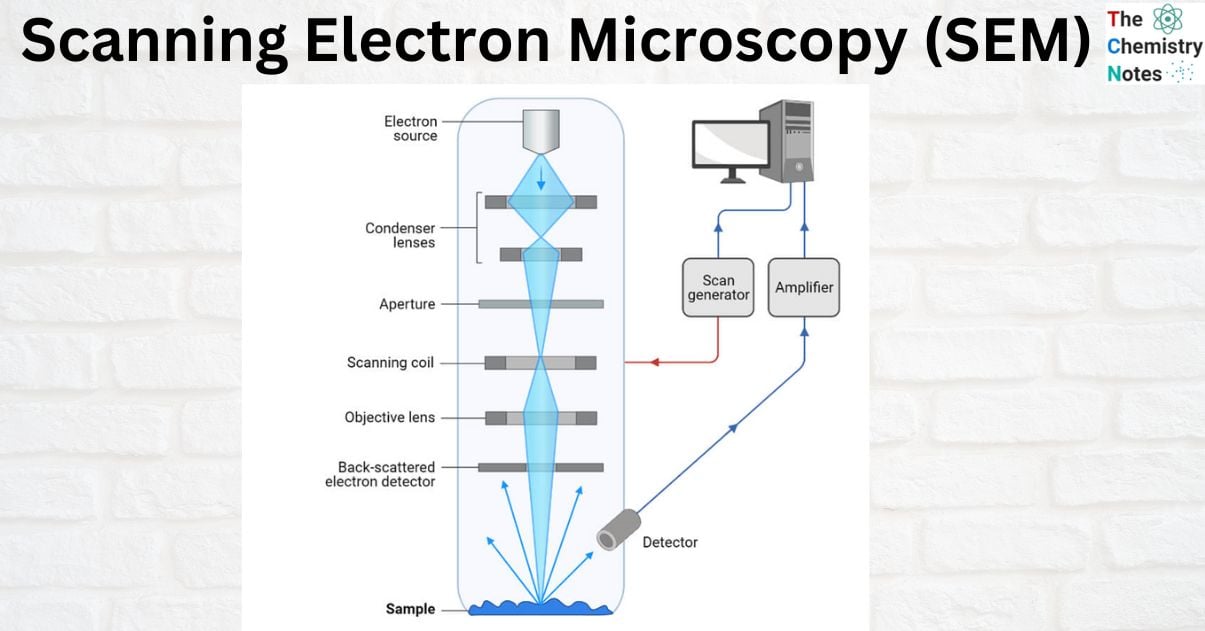
Scanning electron microscopy consists of an electron gun to emit electrons that are focussed into a beam, with a very tiny spot size of ~5 nm. Electrons are accelerated to energy values in the range of a few hundred eV to 50 KeV, then rastered over the surface of the specimen by deflection coils. As the electrons impact and penetrate the surface, a multitude of interactions that result in the emission of electrons and photons from the sample occurs, and Scanning electron microscopy images are formed by collecting the released electrons on a cathode ray tube (CRT) (CRT). Different Scanning electron microscope procedures are classified based on what is later detected and photographed. The principal images produced in Scanning electron microscopy are of three types: secondary electron images, backscattered electron images, and elemental X-ray maps.
Interesting Science Videos
What is Scanning Electron Microscopy?
Scanning electron microscopy (SEM) is such an analytical technique in which a focused beam of high-energy electrons is used, which then generates a range of signals at the surface of the solid specimens it examines.
The signals that are produced as a result of the interactions between the electrons and the sample reveal information about the sample, such as its external morphology, chemical composition, crystalline structure, and orientation of the materials that comprise the sample. In the majority of cases, data are gathered over a certain section of the sample’s surface, and then a two-dimensional image is created to represent the spatial variations in the attributes of the sample.
Conventional Scanning electron microscopy methods (magnifications from 20X to about 30,000X and spatial resolutions of 50 to 100 nm) can be imaged from about 1 cm to 5 microns wide in a scanning mode. The SEM may perform step-by-step analyses of the sample. This is especially useful for identifying chemical compositions (using EDS) and crystalline structures and orientations (using EBSD) in a qualitative or semi-quantitative way. The SEM appears to operate somewhat like the EPMA, and the two instruments have a lot in common in terms of what they may accomplish.
Principle of Scanning Electron Microscopy
When electrons are accelerated through a scanning electron microscopy (SEM), they carry considerable amounts of kinetic energy with them. This energy disappears as a variety of signals created by electron-sample interactions when the incident electrons are decelerated in the solid sample. These signals consist of secondary electrons (which are responsible for the production of SEM images), backscattered electrons (BSE), diffracted backscattered electrons (EBSD), photons (characteristic X-rays that are utilized for elemental analysis and continuum X-rays), visible light, and heat. Samples are typically imaged using either secondary electrons or backscattered electrons, with the former being more useful for displaying sample morphology and topography and the latter being more useful for displaying compositional contrasts in multiphase samples (i.e. for rapid phase discrimination).
Inelastic collisions between incident electrons and electrons in different atomic orbitals (shells) generate X-rays. X-rays of a consistent wavelength (corresponding to the disparity between the energy levels of electrons in various shells for a particular element) are produced as excited electrons decay back to lower energy states. The elements in a mineral can be “excited” by an electron beam, resulting in the emission of distinctive X-rays. Repeated analyses of the same materials are possible because the “non-destructive” nature of Scanning electron microscopy means that the volume of the sample is not diminished due to the x-rays produced by electron interactions.
How is a sample prepared?
Due to the SEM’s dependence on vacuum conditions and electrons to generate an image, the sample must undergo unique preparations. Vacuum conditions necessitate drying out the samples completely. All metals can be used directly from their natural state because of their high conductivity. In order to carry electricity, a tiny layer of conductive material must be applied to every non-metal sample. The tool used for this purpose is termed a “sputter coater.”
An electric field and argon gas control the sputter coater’s coating process. The sample is held in a vacuum-sealed, miniature chamber. In the presence of an electric field, argon gas loses an electron, leaving the atoms with a positive charge. The argon ions are subsequently drawn to the gold foil because of their negative charge. Gold atoms on the foil’s surface are dislodged by the argon ions. A thin gold coating forms as these gold atoms fall and settle on the surface of the sample.
Sample Specification for Scanning electron microscopy
- Since imaging takes place in a dry, high-vacuum (<10-4 pa) environment, samples containing water are difficult to be imaged.
- A conductive sample is required (otherwise, you’ll just get a blurry image of some charging issues in the background and some bright areas where electrons have gathered in the sample).
- Sputtering is used to apply a thin layer of metals like Gold and iron to the non-conductive sample so that it may be photographed.
How does Scanning electron microscopy work?
In a scanning electron microscopy (SEM), an electron beam is released from an electron gun and focused by one or two condenser lenses to a diameter of around 0.4-5 nm. After being bent along the x and y axes by the electron column’s deflection coils, the beam finally makes contact with the sample. This angle shift guarantees a raster scan, which is a rectangular pattern of sample image acquisition. The electron beam loses energy owing to scattering and absorption as it comes into contact with the material.
![Instrumentation of Scanning Electron Microscopy [Image Source: Wikipedia]](https://scienceinfo.com/wp-content/uploads/2023/04/image-86.png)
Signals such as secondary electrons, back-scattered electrons, and distinctive X-rays are generated when the electron beam of a scanning electron microscope interacts with atoms at various depths within the sample. In the Scanning electron microscopy, a separate detector is used to pick up each of these signals. Inelastic scattering of the beam electrons causes low-energy secondary electrons to be expelled from the valence or conduction bands of the sample’s atoms. Electrons from the beam that are reflected through elastic scattering interactions with atoms in the sample are known as back-scattered electrons.
The abundance of various elements in a sample can be determined by measuring the strength of the back-scattered electron signal, which is proportional to the atomic number. When an electron in the inner shell is knocked out by the electron beam, a higher-energy electron can replace the void and release energy, causing the characteristic X-rays to be released. Energy-dispersive X-ray spectroscopy measures the energy or wavelength of these X-rays to determine the amount and distribution of elements in the sample. The distribution of the sample is shown by detecting the electron beam absorbed by the sample. The resulting image is a signal intensity map representing the scanned region of the sample. The SEM may also perform elemental analysis with the addition of EDS detectors.
To capture Scanning electron microscopy images, the sample’s surface must be electrically conductive, and it must also be grounded to prevent static electricity buildup. A conductive adhesive is used to attach samples to the specimen holder. Only conductively mounting the sample to the stage is necessary for sample preparation when working with metals. Electrically conductive compounds like gold, platinum, chromium, or graphite are often applied in extremely thin layers to surfaces of non-conductive materials. The coating is done by sputtering or evaporating substances in a vacuum.
Moreover, a detailed explanation of how a typical Scanning electron microscopy functions is given below:
- The electron gun produces a stream of monochromatic electrons.
- The first condenser lens concentrates the electron beam. The beam is formed and the quantity of current in the beam is restricted by the condenser lens. Condenser aperture and lens work together to filter out high-angle electrons.
- The beam is narrowed by the condenser’s aperture, which causes certain high-angle electrons to be filtered out.
- Typically, the fine probe current knob is used to focus the electrons via the second condenser lens into a narrow, tightly focused, coherent beam.
- Certain high-angle electrons are also removed from the beam by an objective aperture.
- The beam is scanned by a grid of coils, and the scan speed—typically in the microsecond range—determines how long the beam stays at each place.
- The beam is focused on the desired area of the specimen by the objective lens, the final lens.
- A variety of devices are used to detect the radiations produced when the beam interacts with the sample when it hits it.
- These devices count the interactions before the beam advances to its next dwell point, and they show a pixel on a CRT, the brightness of which is dependent on the number of interactions (the greater interactions, the brighter the pixel).
- The scanning speed allows for 30 scans of the complete pattern in one second.
Parts of Scanning Electron Microscope
- Electron source: Here, at a voltage of 1-40kV, electrons are produced using thermal heat. The electrons focus on a beam that can be utilized to create an image and do statistical analysis. Tungsten filaments, lanthanum hexaboride, and field emission guns (FEGs) are the three main electron sources available.
- Lenses: The electron beam from the source is focused by multiple condenser lenses before passing through the column, creating a spot of electrons.
- Scanning coil: Used to redirect the irradiating beam across the surface of the object.
- Detector: There are multiple detectors involved so that the secondary electrons, backscattered electrons, and diffracted backscattered electrons may all be identified and analyzed separately. Detector performance is extremely sensitive to voltage speed and specimen density.
- Display device
- Power supply
- Vacuum system
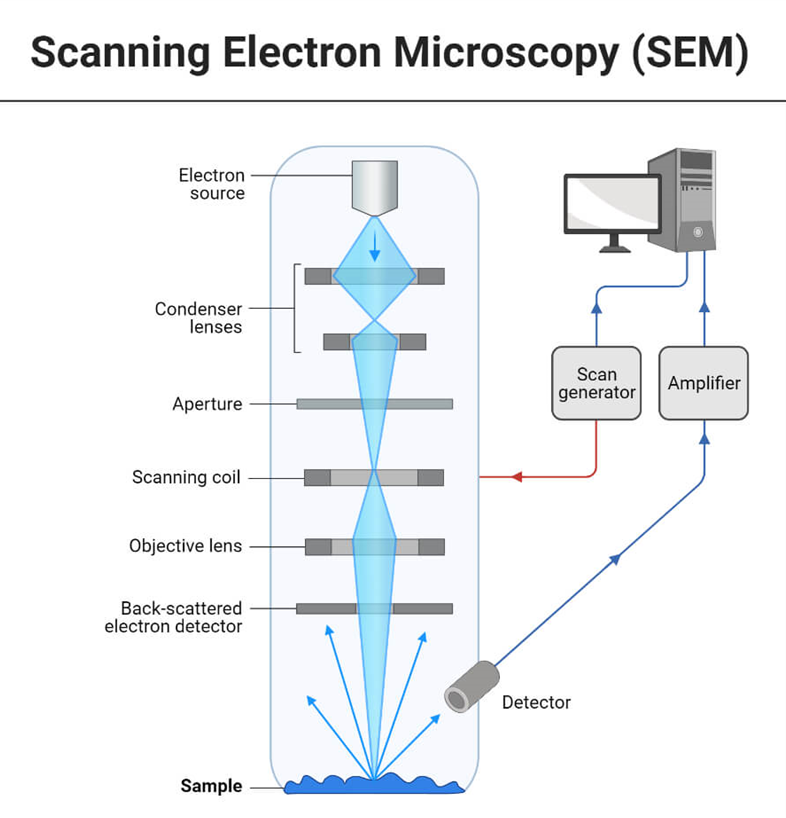
Electron-matter Interaction
When a primary electron with a high amount of energy comes into contact with an atom, the electron will either experience inelastic scattering with the atomic electrons or elastic scattering with the atomic nucleus. When an electron collides with another electron in a way that is not elastic, the first electron gives some of the energy it possesses to the other electron. After the amount of energy that is transmitted is sufficient, the other electron will release themselves from the sample.
The term “secondary electron” refers to an electron that has been emitted from one of the orbitals of the atom that was initially struck by the incoming electron if the energy of the emitted electron is less than 50 eV. SE pictures are extremely sensitive to topographic differences due to the low energy of the secondary electrons.
Backscattered electrons are the high-energy incident electrons that are elastically scattered and essentially possess almost the same energy as the incident or main electrons. Backscattered electrons are created when incident electrons with a high energy are scattered. The higher the atomic number of the sample material, the higher the possibility that the sample will backscatter. Although while backscattering images can’t be utilized for elemental identification, they can nonetheless produce valuable contrasts between areas of the specimen that differ greatly in atomic number, Z. These contrasts can arise between sections of the specimen that have a large range of values.
Hence, the BSE picture is capable of providing both topographic contrast and atomic number contrast in addition to topographic contrast.
An further electron interaction in Scanning electron microscopy is that the primary electron collides with and ejects a core electron from an atom in the sample. The excited atom will decay to its ground state by producing either a distinctive X-ray photon or an Auger electron, both of which have been employed for chemical characterisation.
The energy of the released characteristic X-ray or Auger electrons are particular to the chemistry of the incident atom and consequently examination of them can provide important information on the chemistry of the sample studied. By concentrating the electron beam to precise spots, it is possible to receive localized information on chemical changes. However, it should be remembered that such information on characteristic X-ray (and consequently information on chemistry) is generated from a depth of around a micron and hence the information is averaged over this depth although the beam size could be narrower on a lateral scale.
Combined with chemical analysis capabilities, Scanning electron microscopy not only offers an image of the morphology and microstructures of bulk and nanostructured materials and devices, but may also provide extensive information of chemical composition and distribution.
Imaging
The wavelengths of the electron beam employed and the numerical aperture around the system are what determine the theoretical limit of an instrument’s resolving power. The resolving power, R, of an instrument is defined as:
R = λ/2NA
where;
- NA is a measurement of the condenser or objective’s ability to provide electrons
- λ is the wavelength of the electrons being employed.
The numerical aperture is written on each objective and condenser lens specified.
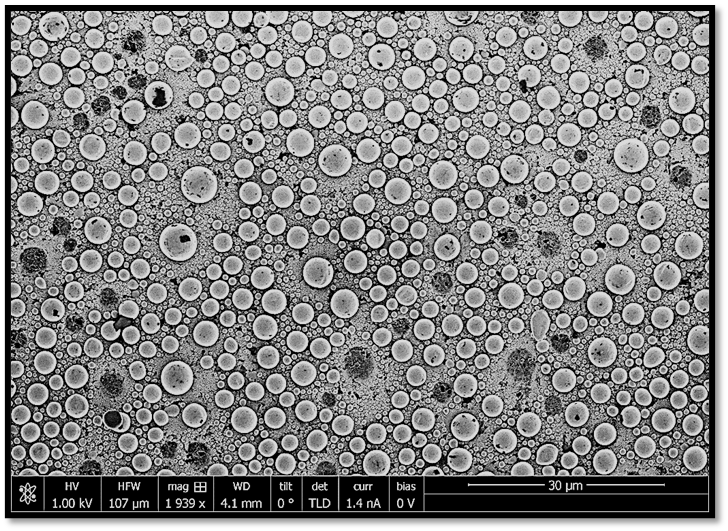
Backscattered and Secondary electrons
Many different kinds of electrons, photons, or irradiations can be produced by the interaction of electrons within a substance. Backscattered (BSE) and secondary (SE) electrons are the two forms of electrons used for imaging in Scanning electron microscopy. After elastic interactions between the beam and the sample, BSEs, which are a part of the main electron beam, are reflected. Conversely, secondary electrons arise from the sample’s atoms and form as a result of inelastic interactions between the sample and the electron beam.
Various forms of information are delivered by BSEs and SEs because they originate from various parts of the sample. There is a clear correlation between atomic number and brightness in BSE images; the higher the atomic number, the brighter the material looks. Greater surface detail can be obtained with SE imaging.
Detected X-rays from electron-matter interactions are commonly employed for elemental analysis in various microscopes. The energy of the X-rays given out by any given material can be thought of as a fingerprint for that material. It is possible to determine the elemental makeup of a sample by measuring the energy of the X-rays that emanate from it.
How electrons are detected?
Separate detectors are used to detect back-scattered electrons and secondary electrons. Back scattered electrons can be detected by positioning solid state detectors above the sample, concentrically to the electron beam. Since various elements reflect a varying number of electrons, backscattered electrons can also be employed to create an image. As a result, different components in a sample will contribute different amounts of energy to the back-scattered electrons.
The distribution of various components in a sample can be shown by constructing a Scanning electron microscopy picture using the intensity of back-scattered electrons and the beam position. Back-scattered electrons can reveal differences in chemical composition by making elements that are heavier and reflect more electrons look brighter in the image. While scanning electron microscopy (SEM) is useful for identifying materials, elemental distribution spectrum (EDS) attachments are required for quantifying elemental abundance.
How to Interpret Data?
Depending on the detector used, several types of Scanning electron microscopy images will be generated, allowing you to examine your material at the nanoscale. Most commonly, secondary electrons emitted from the sample via inelastic scattering are detected by the detector. Grayscale pictures of the topography of the sample are generated by combining the position of the beam with the number of secondary electrons detected, which indicates the signal strength.
Since various elements reflect a varying number of electrons, backscattered electrons can also be employed to create an image. As a result, different components in a sample will contribute different amounts of energy to the back-scattered electrons. The distribution of various components in a sample can be shown by constructing a SEM picture using the intensity of back-scattered electrons and the beam position. Back-scattered electrons can reveal differences in chemical composition by making elements that are heavier and reflect more electrons look brighter in the image. While scanning electron microscopy (SEM) is useful for identifying materials, elemental distribution spectrum (EDS) attachments are required for quantifying elemental abundance.
Information that can be obtained from Scanning electron microscopy
- Topography: The surface characteristics of an object, or “how it looks”; recognizable characteristics are only present within a few manometers.
- Morphology: Shape, size, and arrangement of the component parts of the object that are present on the sample’s surface or have been made visible through chemical etching or grinding; these features are only noticed to a few manometers.
- Composition: The elements and compounds that compose the sample, along with their proportional ratios, are in spaces no larger than 1 micrometer in diameter and depth.
- Crystallographic information: The arrangement of the specimen’s atoms and the degree of order; only applicable to single-crystal particles larger than 20 micrometers.
Advantages of Scanning Electron Microscopy
There may not be any other tool that can compare to the Scanning electron microscopy when it comes to the investigation of solid materials due to its versatility. The scanning electron microscope is indispensable whenever solids must be characterized. Even though geological uses are the primary focus of this contribution, it’s worth noting that these only represent a tiny fraction of the scientific and industrial uses for this equipment. Most Scanning electron microscopy have relatively straightforward interfaces that are “intuitive” to use. Minimal sample preparation is often sufficient for many uses. Data may be acquired quickly for several uses (less than 5 minutes/image for SEI, BSE, and spot EDS analysis). Data from modern SEMs is produced in easily transferable digital formats. Besides these, some of the major advantages are:
- Resolution: Digital images with a resolution of 15 nm can be obtained from this test, providing useful information for defining features of microstructures such cracks, corrosion, grains, and grain boundaries.
- Traceable standard for magnification: Images may be easily analyzed for things like coating thickness, grain size, and particle size because they are all taken against a known reference.
- Chemical analysis: In addition to x-ray line scans and mapping, scanning electron microscopy (SEM) with energy dispersive spectroscopy (EDS) may do qualitative elemental analysis. This information can be utilized for many other analyses, including analyzing product flaws, determining the elemental makeup of foreign components, measuring coating thickness, and measuring grain and particle size.
Limitations of Scanning electron microscopy
Solid samples are required, as is the ability to accommodate them in the microscope’s chamber. The typical upper limit for horizontal dimensions is around 10 cm, whereas the typical upper limit for vertical dimensions is around 40 mm. The vacuum requirements of most equipment require samples to be stable down to the 10-5 to 10-6 torr range. Conventional Scanning electron microscopy are not suited for examining samples that are likely to volatilize at low pressures, including rocks saturated with hydrocarbons, “wet” samples like coal, organic compounds, or swollen clays, and substances likely to decrepitate at low pressure. But there are other “low vacuum” and “environmental” SEMs, and these can investigate many of these samples with no problems.
Many equipment cannot detect elements with atomic numbers lower than 11 (Na), while EDS detectors on Scanning electron microscopy cannot detect very light elements (H, He, and Li). While the solid state x-ray detectors (EDS) used in most SEMs are quick and simple to use, they lack the energy resolution and sensitivity to elements present in low abundances of the wavelength dispersive x-ray detectors (WDS) used in most EPMAs. Samples that are electrically insulating cannot be studied in typical SEMs without first being coated with a conductive material, unless the microscope has a low vacuum mode.
References
- Argast, Anne and Tennis, Clarence F., III, 2004, A web resource for the study of alkali feldspars and perthitic textures using light microscopy, scanning electron microscopy and energy dispersive X-ray spectroscopy, Journal of Geoscience Education 52, no. 3, p. 213-217.
- McMullan, D. Scanning electron microscopy 1928-1965. Scanning 17, 175–185 (2006).
- Smith, K. C. A. & Oatley, C. W. The scanning electron microscope and its fields of application. Br. J. Appl. Phys. 6, 391–399 (1955).
- Beane, Rachel, 2004, Using the Scanning Electron Microscope for Discovery Based Learning in Undergraduate Courses, Journal of Geoscience Education, vol 52 #3, p. 250-253
- https://chemistnotes.com/physical/scanning-electron-microscopy-principle-and-applications/
- https://microbenotes.com/scanning-electron-microscope-sem/
- Antonovsky, A. (1984). “The application of colour to SEM imaging for increased definition”. Micron and Microscopica Acta. 15 (2): 77–84. doi:10.1016/0739-6260(84)90005-4
- Goldstein, G. I.; Newbury, D. E.; Echlin, P.; Joy, D. C.; Fiori, C.; Lifshin, E. (1981). Scanning electron microscopy and x-ray microanalysis. New York: Plenum Press. ISBN 978-0-306-40768-0.
- Oatley CW, Nixon WC, Pease RFW (1965) Scanning electron microscopy. Adv Electronics Electron Phys
- Stokes, Debbie J. (2008). Principles and Practice of Variable Pressure Environmental Scanning Electron Microscopy (VP-ESEM). Chichester: John Wiley & Sons. ISBN 978-0470758748.