Supramolecular chemistry is the branch of chemistry concerned with the investigation of complex molecular systems composed of several discrete chemical components. Because these multicomponent entities are formed by reversible interactions, they can dissociate and reform in response to specific chemical or environmental stimuli. The combination of these components results in the formation of new entities with distinct properties that frequently behave in entirely novel and unexpected ways. The
The resulting supramolecular phenomena could be as simple as crystal formation from a saturated solution or as complex as a ribosomal translation of messenger RNA into a protein. Finally, supramolecular chemists take simple molecules and use non-covalent forces to assemble them into highly functional nanoscale objects.
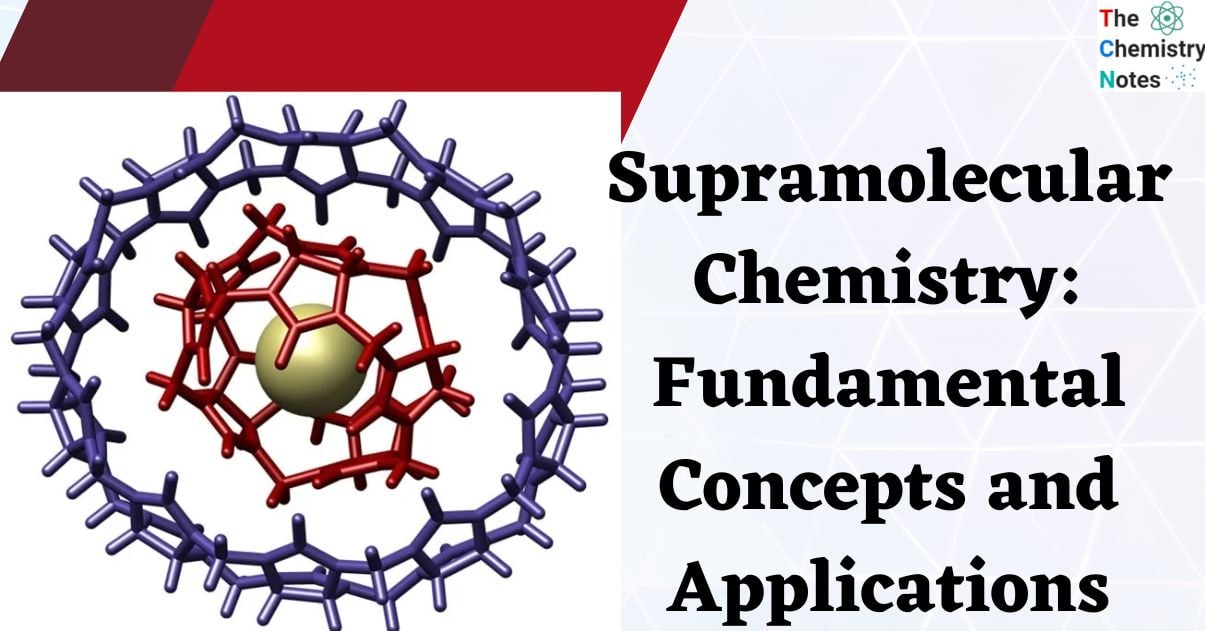
Interesting Science Videos
What is Supramolecular Chemistry?
Supramolecular Chemistry: The study of systems involving aggregates of molecules or ions held together by non-covalent interactions, such as electrostatic interactions, hydrogen bonding, dispersion interactions, and solvophobic effect.
Supramolecular chemistry is a multidisciplinary field that has an impact on many other disciplines, including the traditional areas of organic and inorganic chemistry.
Chemistry is required to synthesize the precursors for a supermolecule, followed by physical chemistry and computational modeling to understand the properties of complex supramolecular systems. Supramolecular concepts are used extensively in biological chemistry, and a certain level of technical knowledge is required to apply supramolecular systems to real-world applications such as the development of nanotechnological devices.
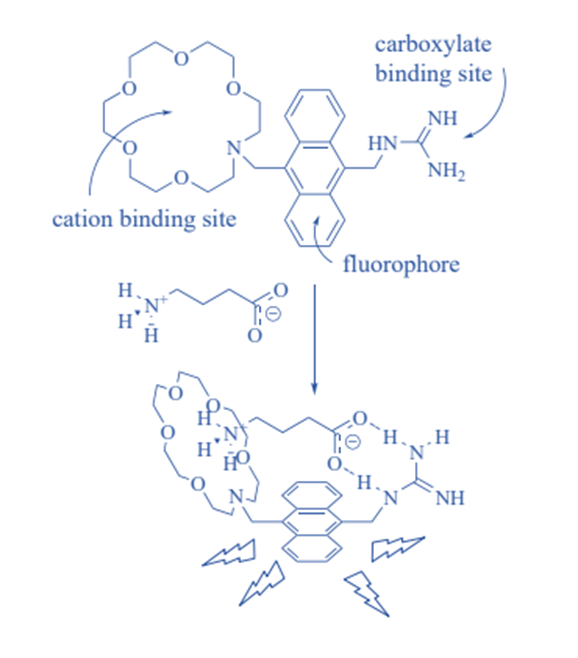
History and Development of the Concept of Supramolecular Chemistry
Johannes Diderik van der Waals proposed the existence of intermolecular forces in 1873. Supramolecular chemistry, on the other hand, has philosophical roots in the work of Nobel laureate Hermann Emil Fischer. Fischer proposed in 1890 that interactions between an enzyme and its substrate resembled a “lock and key,” a concept that has since been useful in explaining molecular recognition and host-guest chemistry.
The application of these principles aided in the development of a better understanding of the structures of various biological macromolecules and processes. Concurrently, chemists began to recognize and study synthetic structures involving noncovalent interactions, such as micelles and microemulsions.
Chemists eventually applied these concepts to synthetic systems. Charles J. Pedersen’s synthesis of crown ethers in the 1960s was a game changer. Throughout the 1980s, research in the field advanced at a rapid pace, with concepts such as mechanically interlocked molecular architectures emerging.
Donald J. Cram, Jean-Marie Lehn, and Charles J. Pedersen were awarded the Nobel Prize in Chemistry in 1987 for their work in supramolecular chemistry. The development of selective “host-guest” complexes, in which a host molecule recognizes and selectively binds to a specific guest, was cited as an important contribution.
Supramolecular chemistry advanced further in the 1990s, with researchers like James Fraser Stoddart developing molecular machinery and highly complex self-assembled structures. To increase functionality, electrochemical and photochemical motifs were integrated into supramolecular systems during this time period. In addition, research into synthetic self-replicating systems and molecular information processing devices has begun. The subject was also heavily influenced by the emerging science of nanotechnology, with building blocks such as fullerenes, nanoparticles, and dendrimers becoming involved in synthetic systems.
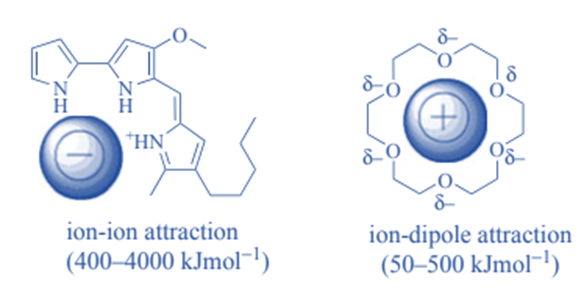
Supramolecular Chemistry: Fundamental Concepts and Literature
The following are important concepts developed over the last few decades that have played an important role in understanding and developing several areas of application.
Molecular self-assembly
Molecular self-assembly is the folding of individual molecules (such as polypeptides) or the formation of structures involving two or more molecules via noncovalent interactions. As a result, the process can be classified as intramolecular or intermolecular. The molecules are said to “self-assemble” because the structures form based on the inherent properties of the molecules involved, with no external management (other than the provision of a suitable environment).
Large structures such as micelles, membranes, vesicles, and liquid crystals can be built using molecular self-assembly. It is critical in crystal engineering. Perhaps the best-known intermolecular self-assembling structure in biological systems is naturally occurring DNA, which exists in a double helical form.
Molecular recognition and complexation
The specific binding of a “guest” molecule to a complementary “host” molecule to form a “host-guest complex” is referred to as molecular recognition. The terms “host” and “guest” are often assigned arbitrarily to the molecular species. Noncovalent interactions allow the molecules to recognize one another. This field’s key applications include the development of molecular sensors and catalysis.
Non-covalent bonding such as hydrogen bonding, metal coordination, hydrophobic forces, van der Waals forces, – interactions, halogen bonding, electrostatic and/or electromagnetic effects are used to interact between host and guest molecules. Aside from these direct interactions, the solvent can play a significant indirect role in driving molecular recognition in solution. Molecular complementarity exists between the host and the guest in molecular recognition.
Molecular recognition plays an important role in biological systems and is observed in between receptor ligand, antigen-antibody, DNA-protein, sugar-lectin, RNA-ribosome, etc. The antibiotic vancomycin, which selectively binds to peptides with terminal D-alanyl-D-alanine in bacterial cells via five hydrogen bonds, is an important example of molecular recognition. Vancomycin is lethal to bacteria once it has bound to them.
specific peptides that cannot be used to build the bacteria’s cell wall Recent research suggests that molecular recognition elements can be synthesized at the nanoscale, eliminating the need for naturally occurring molecular recognition elements in the development of small molecule sensing tools.
Template-directed synthesis
With reactive species, molecular recognition and self-assembly can be used to pre-organize a system for a chemical reaction (to form one or more covalent bonds). It could be considered a subset of supramolecular catalysis. Noncovalent bonds between the reactants and a “template” keep the reactants’ reactive sites close together, allowing for the desired chemistry. This method is especially useful when the desired reaction conformation is thermodynamically or kinetically unlikely, such as when preparing large macrocycles. This pre-organization also serves other purposes, such as reducing side reactions, lowering the reaction activation energy, and producing desired stereochemistry.
Following the reaction, the template may be left in place, forcibly removed, or “automatically” decomplexed due to the different recognition properties of the reaction product. The template could be as simple as a single metal ion or very complex.
Mechanically-interlocked molecular architectures
Mechanically interlocked molecular architectures are made up of molecules that are only linked by their topology. There may be some non-covalent interactions between the various components, but no covalent bonds. The efficient synthesis of the compounds is dependent on supramolecular chemistry and template-directed synthesis. Catenanes, rotaxanes, molecular knots, molecular Borromean rings, and ravels are examples of mechanically interlocked molecular architectures.
The mechanically interlocked molecular architecture of molecular Borromean rings is one example.
Three macrocycles are linked in such a way that any macrocycle that is broken allows the others to disassociate. They are the smallest Borromean rings found. Borromeate is composed of three interconnected macrocycles formed by the reaction of 2,6-di-formyl pyridine and diamine compounds complexed with zinc.
Dynamic Covalent Chemistry
The focus of dynamic covalent chemistry is synthesizing large complex molecules from simple one of large complex molecules from simple ones is the focus of dynamic covalent chemistry. Out of many products, only one is captured in this case from a reversible reaction under thermodynamic reaction control. The concept of dynamic covalent chemistry was expanded upon in the formation of specific molecular Borromean rings.
It is illustrated in an illustrative example involving the irreversible highly diluted reaction of a diol with chlorobromo methane in the presence of sodium hydride. The dimer, on the other hand, is part of a series of equilibria between polyacetal macrocycles of varying sizes caused by acid-catalyzed (triflic acid) trans-acetalization.
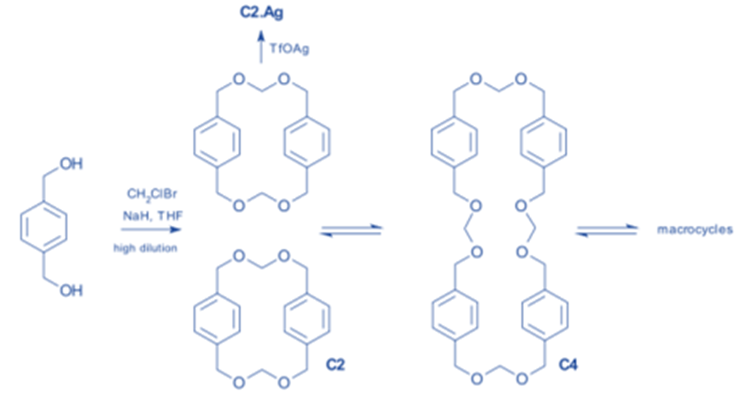
Techniques for Molecular Imprinting
Molecular imprinting is a technique for creating a host from small molecules by using a suitable molecular species as a template. Molecularly imprinted materials are prepared using a template molecule and functional monomers that assemble around the template and subsequently get crosslinked to each other. The template molecule is extracted from the matrix under specific conditions, leaving a cavity complementary in size and shape to the template.
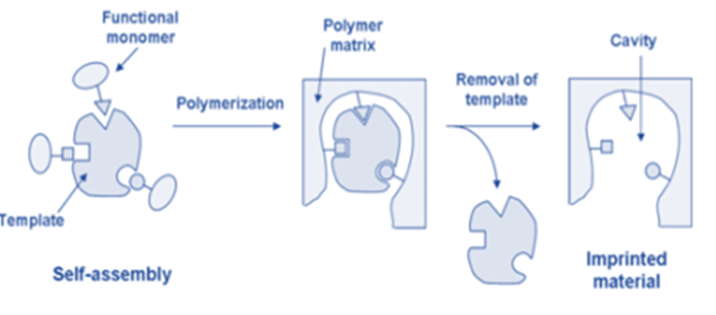
This method is based on the “lock and key” model, which is used by enzymes for substrate recognition. An enzyme’s active binding site has a distinct geometric structure that is ideal for binding a substrate. A substrate with the correct shape for the binding site is recognized by selectively binding to the enzyme, whereas an incorrectly shaped molecule that does not fit the binding site is not recognized.
Molecular Machine Concepts
The molecular machine concept (also known as the nanomachine) has biological applications. Molecular Machine Concepts It is defined as any discrete number of molecular components that respond to specific stimuli (input) by producing quasi-mechanical movements (output). The expression is often more generally applied to molecules that mimic functions at the macroscopic level.
Molecular machines are classified into two types: synthetic and biological. A potentially important branch of chemistry and nanotechnology is molecular systems capable of shifting a chemical or mechanical process away from equilibrium. Because the gradient produced by this process can perform useful work, these systems are, by definition, examples of molecular machinery.
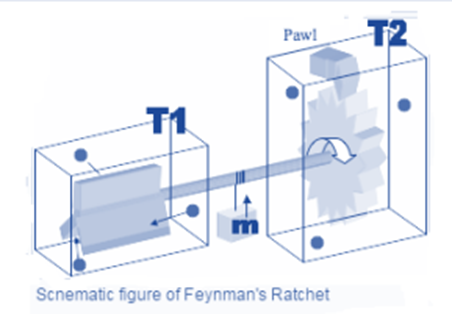
Biomimetics
Biomimetics, also known as biomimicry, is the use of knowledge derived from natural models or systems to solve real-world or scientific problems. The terms biomimetics and biomimicry are derived from the Greek words bios (life) and mmsis (mimicry) (imitation). Natural selection has resulted in the evolution of well-adapted structures and materials in living organisms over geological time. Biomimetics has given rise to new technologies at the macro and nanoscales that are inspired by biological solutions. Throughout history, humans have looked to nature for solutions to problems. Nature has solved engineering issues such as as self-healing abilities, environmental tolerance and resistance, hydrophobicity, self-assembly, and solar energy harvesting. Many synthetic supramolecular systems are designed to mimic biological system functions. Photo-electrochemical systems, catalytic systems, protein design, and self-replication are a few examples.
Building blocks of Supramolecular chemistry
Supramolecular systems are rarely created from scratch. Chemists, on the other hand, have a variety of well-studied structural and functional building blocks from which to construct larger functional architectures. Many of these building blocks exist as families of similar units from which to select the analog with the families of similar units from which to select analog with the exact desired properties.
Motifs of synthetic recognition
- Crown ether binding with metal or ammonium cations is commonly used in supramolecular chemistry.
- Carboxylic acid dimer formation and other simple hydrogen bonding interactions
- Bipyridinium’s pi-pi charge-transfer interactions with dioxyarenes or diaminoarenes have been extensively used in the construction of mechanically interlocked systems and in crystal engineering.
- The complexation of bipyridines or tripyridines with ruthenium, silver, or other metal ions is extremely useful in the formation of complex architectures composed of many individual molecules.
- Complexation of porphyrins or phthalocyanines with metal ions provides access to catalytic, photochemical, and electrochemical properties, as well as complexation. These units are common in nature.
Macrocycles
- Macrocycles are extremely useful in supramolecular chemistry because they contain entire cavities that can completely surround guest molecules and can be chemically modified to fine-tune their properties.
- Cyclodextrins, calixarenes, cucurbiturils, and crown ethers are all easily synthesized and thus suitable for use in supramolecular systems.
- To provide more tailored recognition properties, more complex cyclophanes and cryptands can be synthesized.
Structural elements
Many supramolecular systems necessitate appropriate spacing and conformations between their component molecules. The construction of these systems necessitates the use of readily available structural units.
- Polyether chains, biphenyls, triphenyls, and simple alkyl chains are common spacers and connecting groups. The chemistry involved in the formation and connection of these units is well understood.
- Nanoparticles, nanorods, fullerenes, and dendrimers are structures and encapsulation units with nanometer dimensions.
- Surfaces can be used as scaffolds to build complex systems as well as to interface electrochemical systems with electrodes. Regular surfaces can be used to make self-assembled monolayers and multilayers.
Photochemically or electrochemically active units
- Porphyrins and phthalocyanines have highly tunable photochemical and electrochemical activity, as well as the ability to form complexes.
- When exposed to light, photochromic and photoisomerizable groups change their shapes and properties (including binding properties).
- Because tetrathiafulvalene (TTF) and quinones have multiple stable oxidation states, they can be switched using redox chemistry or electrochemistry.
- Other units, such as benzidine derivatives, viologen groups, and fullerenes, have also been used in supramolecular electrochemical devices.
Biologically derived units
- The extremely strong complexation of avidin and biotin is important in blood clotting and has been used as a recognition motif in the development of synthetic systems.
- Enzyme-cofactor binding has been used to create modified enzymes, electrically contacted enzymes, and even photoswitchable enzymes.
- DNA has been used in synthetic supramolecular systems as both a structural and functional unit.
Applications of Supramolecular Chemistry
Materials science and technology
Supramolecular chemistry, in particular molecular self-assembly processes, has been used to create new materials. Because large structures are composed of small molecules that require fewer steps to synthesize, they can be easily accessed using bottom-up synthesis. Supramolecular chemistry underpins the majority of bottom-up approaches in nanotechnology.
Catalysis
The design and study of catalysts and catalysis is a major application of supramolecular chemistry. Noncovalent interactions are extremely important in catalysis, binding reactants into reaction-suitable conformations and lowering the reaction’s transition state energy. Supramolecular catalysis is a subset of template-directed synthesis. Catalysis also employs encapsulation systems such as micelles and dendrimers to create microenvironments suitable for reactions (or reaction steps) that would otherwise be impossible.
Medicine
Supramolecular chemistry aids in the development of new pharmaceutical therapies by providing insight into the interactions at drug binding sites. Supramolecular chemistry, which provides encapsulation and targeted-release mechanisms, has also made significant advances in the field of drug delivery. Furthermore, supramolecular systems have been developed to disrupt protein-protein interactions that are critical to cellular functions.
Storage and processing of data
Supramolecular chemistry has been used to demonstrate molecular computation functions. In many cases, photonic or chemical signals have been used in these components, but supramolecular signal transduction devices have demonstrated electrical interfacing of these units. Molecular switches with photochromic and photoisomerizable units, electrochromic and redox-switchable units, and molecular motion have all been used to store data. On a conceptual level, synthetic molecular logic gates have been demonstrated. Semi-synthetic DNA computers have completed full-scale computations.
Environmental chemistry
Supramolecular chemistry research has enabled the development of solid-state reactions governed by noncovalent bonding. Such processes are highly desirable because they reduce the need for solvents in chemical production. These approaches are thus part of what is known as green chemistry.
High-Tech Devices
Supramolecular chemistry is frequently pursued in order to develop new functions that cannot be produced by a single molecule. Magnetic properties, light responsiveness, self-healing polymers, synthetic ion channels, molecular sensors, and other functions are also included. Supramolecular research has been used to create high-tech sensors, radioactive waste treatment processes, and contrast agents for CAT scans.
References
- Supramolecular Chemistry-Concepts and Applications, International Journal of Science and Research (IJSR) – Ajay Kumar Manna Paper ID: 29031502
- https://www.newworldencyclopedia.org/entry/supramolecular_chemistry
- Cragg, Peter J. 2005. A Practical Guide to Supramolecular Chemistry. Chichester: John Wiley. ISBN 0470866543
- Schneider, Hans-Jörg and Anatoly K. Yatsimirsky. 2000. Principles and Methods in Supramolecular Chemistry. Chichester: John Wiley. ISBN 0471972533
- Steed, Jonathan W., David R. Turner, and Karl J. Wallace. 2007. Core Concepts in Supramolecular Chemistry and Nanochemistry. Chichester, England: John Wiley. ISBN 9780470858677
- Lehn, J. M. (1990). “Perspectives in Supramolecular Chemistry—From Molecular Recognition towards Molecular Information Processing and Self-Organization”. Angewandte Chemie International Edition in English. 29 (11): 1304–1319. doi:10.1002/anie.199013041