The periodic table elements are frequently classified into four groups: (1) main group elements, (2) transition metals, (3) lanthanides, and (4) actinides. Transition elements are elements found in Groups 3-12 of the periodic table (old groups IIA-IIB). The word refers to the fact that the d sublevel being filled has a lower principal energy level than the s sublevel before it. Transition metals are elements that have (or can easily create) partially filled d orbitals.
The lanthanides and actinides at the bottom of the table are frequently referred to as the inner transition metals because their atomic numbers fall between the first and second elements in the transition metals’ last two rows.
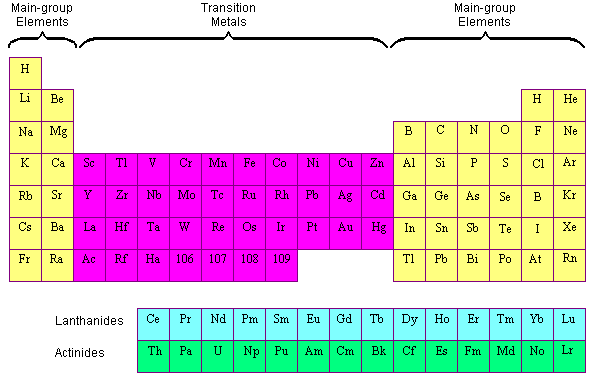
What are transition metals?
The transition metals are metallic elements that act as a link between the two sides of the table. The transition elements are found in the d block of the Periodic Table, between Groups 2 and 13. However, not all d-block elements are classified as transition elements.
A transition element is a d-block element that forms one or more stable ions with an incomplete d subshell. It derives from the Latin transientum, which means ‘passing over,’ and denotes a movement from one location to another.
Transition metals are a class of elements that bridge the gap between two sides of the periodic table.
We do not define Sc and Zn as transition elements.
Scandium forms only one ion (Sc3+) and this has no electrons in its 3d subshell – the electronic configuration of Sc3+ is (Ar) 3d0 4s0.
Zinc forms only one ion (Zn2+) and this has a complete 3d subshell – the electronic configuration of Zn2+ is (Ar) 3d10 4s0.
Electronic Configuration of Transition Metals
The first two rows of transition elements, together with their electric configurations, are tabulated below.
It can be noted that in some of these elements, the configuration of electrons corresponds to (n-1)d5 ns1 or (n-1)d10 ns1 because of the stability provided by the half-filled or completely filled electron orbitals.
Transition Elements | Atomic Number | Electronic Configuration |
Sc | 21 | [Ar] 3d1 4s2 |
Ti | 22 | [Ar] 3d2 4s2 |
V | 23 | [Ar] 3d3 4s2 |
Cr | 24 | [Ar] 3d5 4s1 |
Mn | 25 | [Ar] 3d5 4s2 |
Fe | 26 | [Ar] 3d6 4s2 |
Co | 27 | [Ar] 3d7 4s2 |
Ni | 28 | [Ar] 3d8 4s2 |
Cu | 29 | [Ar] 3d10 4s1 |
Zn | 30 | [Ar] 3d10 4s2 |
Y | 39 | [Kr] 4d1 5s2 |
Zr | 40 | [Kr] 4d2 5s2 |
Nb | 41 | [Kr] 4d4 5s1 |
Mo | 42 | [Kr] 4d5 5s1 |
Tc | 43 | [Kr] 4d5 5s2 |
Ru | 44 | [Kr] 4d7 5s1 |
Rh | 45 | [Kr] 4d8 5s1 |
Pd | 46 | [Kr] 4d10 |
Ag | 47 | [Kr] 4d10 5s1 |
Cd | 48 | [Kr] 4d10 5s2 |
The 4s subshell is generally occupied in transition element atoms, while the remaining electrons occupy orbitals in the 3d subshell. The exceptions are chromium and copper atoms. In the 4s subshell, chromium atoms have only one electron. The remaining five electrons are organized in the 3d subshell so that one electron occupies each orbital. Copper atoms have one electron in the 4s subshell as well. The remaining ten electrons are organized in the 3d subshell so that two electrons fill each orbital.
Oxidation State
All of the transition elements are metals. Their atoms, like all metals, prefer to lose electrons, resulting in positively charged ions. Each transition metal, however, can create more than one ion. Transition elements exhibit a wide variety of oxidation states in their compounds.
For example, manganese shows all the oxidation states from +2 to +7 in its compounds. However, some elements exhibit few oxidation states, like Sc, and Zn. The lower oxidation states exhibited by these elements is attributed to the fact that they have few electrons to lose (hence, fewer orbitals to share electron with others) for higher valence for example Zn. The variable oxidation states of transition elements arise mainly out of incomplete filling of d orbitals in such a way that their oxidation states differ from each other by unity. Copper’s common ions, for example, are Cu+ and Cu2+. Transition metals are defined as having varying oxidation states. The resultant ions are frequently of distinct colors.
Element | Most common oxidation states |
Titanium (Ti) | +3, +4 |
Vanadium (V) | +2, +3, +4, +5 |
Chromium (Cr) | +3, +6 |
Manganese (Mn) | +2, +4, +6, +7 |
Iron (Fe) | +2, +3 |
Cobalt (Co) | +2, +3 |
Nickel (Ni) | +2 |
Copper (Cu) | +1, +2 |
Because of the existence of variable oxidation states, the names of compounds containing transition elements must include the oxidation number, for example, manganese (IV) oxide and cobalt (II) chloride.
Ions and Oxidation States
When transition elements combine to create ions, their atoms lose electrons from the 4s subshell first, followed by electrons from the 3d subshell.
V atom = 1s2 2s2 2p6 3s2 3p6 3d3 4s2; V3+ ion = 1s2 2s2 2p6 3s2 3p6 3d2 4s0
Fe atom = 1s2 2s2 2p6 3s2 3p6 3d6 4s2; Fe3+ ion = 1s2 2s2 2p6 3s2 3p6 3d5 4s0
Cu atom = 1s2 2s2 2p6 3s2 3p6 3d6 3d10 4s1; Cu2+ ion = 1s2 2s2 2p6 3s2 3p6 3d6 3d9 4s0
The most common oxidation state is +2, which is generated when the atoms lose their two 4s electrons.
The highest oxidation number of the transition elements at the beginning of the row involves all of the atoms’ 4s and 3d electrons. Vanadium, for example, has a maximum oxidation state of +5, involving two 4s electrons and three 3d electrons. From iron onwards, the +2 oxidation state predominates since 3d electrons become progressively difficult to remove as the nuclear charge grows over time.
The higher oxidation states of the transition elements are found in complex ions or in compounds formed with oxygen or fluorine. Common examples are the chromate (VI) ion, CrO4 2–, and the manganate (VII) ion, MnO4 –.
Physical Properties of the Transition Metals
Because their electrical structures differ from those of other transition metals, the elements zinc, cadmium, and mercury are not considered transition elements. However, the characteristics of the remaining d-block elements are somewhat similar, and this similarity can be seen along each row of the periodic table. The properties of the transition elements are listed below.
- These elements combine to generate colored compounds and ions. This color is explained by the electron d-d transition.
- The energy gap between these elements’ potential oxidation states is relatively small. As a result, the transition elements have a wide range of oxidation states.
- Because of the unpaired electrons in the d orbital, these elements create a large number of paramagnetic compounds.
- These elements can be bound by a wide range of ligands. As a result, transition elements generate a wide range of stable complexes.
- These elements have a high charge-to-radius ratio.
- When compared to other elements, transition metals are hard and have comparatively high densities.
- Because delocalized d electrons participate in metallic bonding, the boiling and melting temperatures of these elements are high.
- The metallic bonding of the delocalized d electrons also makes the transition elements effective electrical conductors.
The transition elements commonly have physical properties that are typical of most metals:
- they have high melting points
- they have high densities
- they are hard and rigid, and so are useful as construction materials
- they are good conductors of electricity and heat
Periodic Trend of Transition Elements
The elements in the second and third rows of the Periodic Table exhibit expected gradual variations in characteristics throughout the table from left to right. Electrons in the outer shells of these elements’ atoms have little shielding effect, resulting in an increase in effective nuclear charge due to the addition of protons in the nucleus. As a result, the consequences on atomic characteristics are as follows: lower atomic radius, increased first ionization energy, enhanced electronegativity, and greater nonmetallic character. This pattern continues until one reaches calcium (Z=20). At this point, there is an abrupt break. The physical and chemical properties of the next ten elements, known as the first transition series, are eerily identical.
This broad property similarity has been explained by the series’ relatively tiny difference in effective nuclear charge. This happens because each extra electron enters the penultimate 3d shell, which acts as a barrier between the nucleus and the outer 4s shell.
Atomic Radius and Ionic Radius
The atomic and ionic radii of the transition elements fall from group 3 to group 6 due to the inadequate shielding provided by the small number of d-electrons. Those in groups 7 and 10 have atomic radii that are somewhat similar, but those in groups 11 and 12 have bigger radii. This is due to electron-electron repulsions canceling out the nuclear charge.
As one progresses through the group, the atomic and ionic radii of the elements rise. The presence of a greater number of subshells can explain the rise in radius.
Ionization Energy
The amount of energy required to remove a valence electron from an element is referred to as its ionization enthalpy. The larger the effective nuclear charge acting on electrons, the greater the element’s ionization potential. As a result, the ionization enthalpies of transition elements are often higher than those of s-block elements.
In some ways, an element’s ionization energy is connected to its atomic radius. Atoms with lower radii have higher ionization enthalpies than those with larger radii. As one moves down the row, the ionization energy of the transition metals increase (due to the increase in atomic number).
The first ionization energy, the atomic radius, and the ionic radius of transition elements do not vary much as we go across the first row.
Elements | Ionization Energy | Atomic Radius | Ionic Radius |
Titanium (Ti) | 661 | 0.132 | Ti2+ 0.090 |
Vanadium (V) | 648 | 0.112 | V3+ 0.074 |
Chromium (Cr) | 653 | 0.117 | Cr3+ 0.069 |
Manganese (Mn) | 716 | 0.117 | Mn2+ 0.080 |
Iron (Fe) | 762 | 0.116 | Fe2+ 0.076 |
Cobalt (Co) | 757 | 0.116 | Co2+ 0.078 |
Nickel (Ni) | 736 | 0.115 | Ni2+ 0.078 |
Copper (Cu) | 745 | 0.117 | Cu2+ 0.069 |
Catalytic activity
Transition metals make excellent homogeneous or heterogeneous catalysts; iron, for example, is the catalyst for the Haber process. Vanadium(V) oxide is used in the contact process, nickel is utilized in the production of margarine, and platinum is used to accelerate the production of nitric acid. Because they may produce a variety of oxidation states, they can form new compounds throughout a reaction, providing an alternative route with lower total activation energy.
Colored compounds
Color is seen by humans as different frequencies of electromagnetic energy in the visible part of the electromagnetic spectrum. Different hues occur from changes in the composition of light after it has been reflected, transmitted, or absorbed by a substance. Transition metals, due to their structure, produce a wide range of colored ions and complexes.
Color can also differ amongst ions of the same element – MnO4 (Mn in oxidation state 7+) is purple, whereas Mn2+ is pale-pink.
Due to changes in the energy of the d orbitals, coordination by ligands can play a role in defining color in a transition compound. Ligands reduce orbital degeneracy and divide them into higher and lower energy groups. Because electromagnetic radiation is only absorbed if it has energy equivalent to that gap, the energy gap between the lower and higher energy orbitals will affect the color of the light that is absorbed. Some electrons are propelled to a higher energy orbital when a ligated ion absorbs light. Different colors are perceived because different frequencies of light are absorbed.
Cause of color of a Complex
- the metal ion’s nature, specifically the number of electrons in the d orbitals
- the type of the ligands surrounding the metal ion, and the arrangement of the ligands around the metal ion (for example, geometric isomers can display distinct hues). The bigger the energy difference between the divided high and low 3d groups, the stronger the ligands.
- Because the 3d orbitals are full – no electrons can migrate up to the upper group – the complex ion generated by the d block element zinc (though not strictly a transition element) is colorless.
Transition Metal Ion | Color |
Co2+ | pink |
Cu2+ | blue-green |
Fe2+ | olive green |
Ni2+ | bright green |
Fe3+ | brown to yellow |
CrO42- | orange |
Cr2O72- | yellow |
Ti3+ | purple |
Cr3+ | violet |
Mn2+ | pale pink |
Zn2+ | colorless |
Uses of Transition Metals
- Transition metals offer a wide range of applications due to their characteristics. They can be found in electronics, building materials, and other places. Here are some of their most common uses:
- Because aluminum is lightweight and non-toxic, it is utilized not only in the production of vehicle and aircraft parts but also in the production of cans and foil for food packaging.
- Iron is utilized in construction materials such as bridges, ships, and building structures. Because of its tremendous strength and low cost. In reality, iron accounts for 90% of global metal output.
- Because of its high electrical conductivity, copper is employed in electrical cables.
- Powdered titanium is used in the pyrotechnics sector, such as fireworks since it generates brilliantly blazing particles.
- Tungsten is utilized in the filaments of light bulbs and X-ray tubes.
References
- J. D. Lee, Concise Inorganic Chemistry, 5th Edition, John Wiley and Sons. Inc. 2007.
- F. A. Cotton, G. Wilkinson & C. Gaus, Basic Inorganic Chemistry, 3 rd Edition, John Wiley & Sons (Asia), Pvt., Ltd., 2007.
- Cotton, F. Albert; Wilkinson, Geoffrey (1988). Advanced Inorganic Chemistry (5th ed.). New York: Wiley-Interscience. ISBN 0-471-84997-9.
- https://www.britannica.com/science/transition-metal
- https://byjus.com/chemistry/transition-elements/
- https://chem.libretexts.org/Bookshelves/General_Chemistry/Map%3A_General_Chemistry_(Petrucci_et_al.)/23%3A_The_Transition_Elements/23.1%3A_General_Properties_of_Transition_Metals
- https://www.chemeurope.com/en/encyclopedia/Transition_metal.html
- https://www.vedantu.com/chemistry/transition-elements