A material’s ultimate tensile strength (UTS), often known as tensile strength, is the highest stress it can bear before breaking or collapsing under tension. Tensile strength is a synonym for ultimate tensile strength. Ultimate tensile strength can occasionally be expressed as the letters “UTS” or “TS.” The ultimate tensile stress felt before the material totally fails is the property that is described by all the phrases combined. It’s an essential characteristic that helps predict a material’s or component’s behavior under load. Units of force per unit area, such as Pascals (Pa) or pounds per square inch (psi), are commonly used to express the UTS.
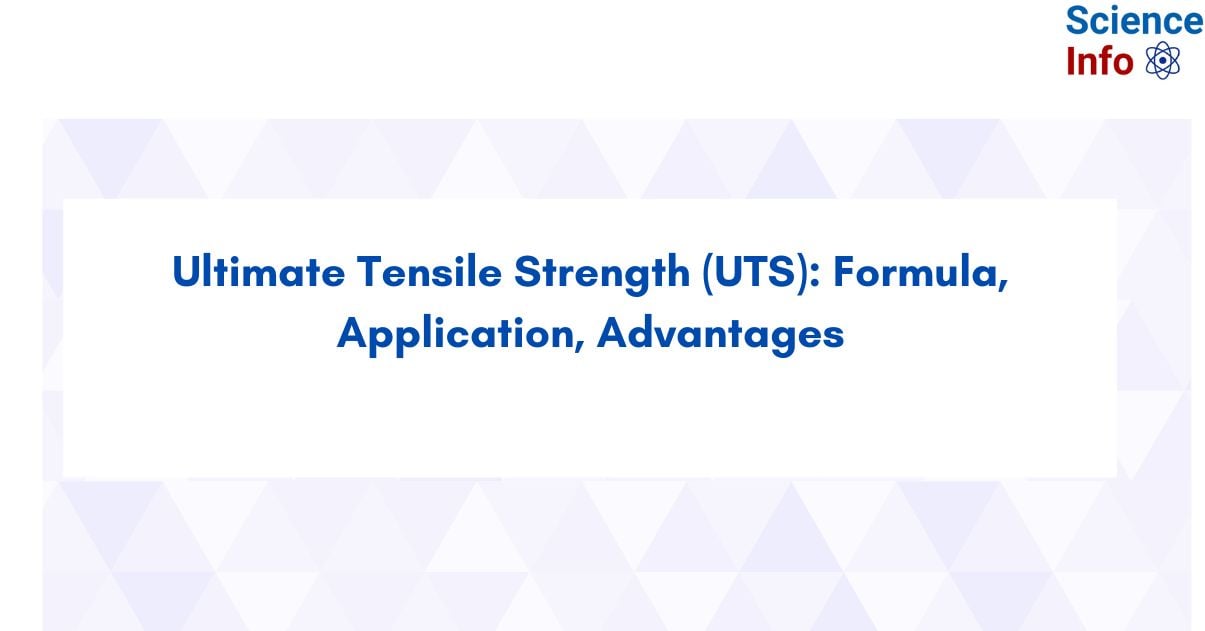
Interesting Science Videos
What is Ultimate Tensile Strength?
- The maximum load that a material can withstand before breaking is known as its ultimate tensile strength.
- A tensile testing machine holds a test specimen until it breaks in order to determine a material’s tensile strength.
- Calculating the material properties can be done using the force applied and the rate of elongation. One can calculate a material’s final tensile strength by dividing the force needed to break it by the material’s initial cross-sectional area.
- Tensile testing is used to determine tensile strength, including the UTS. A tensile test is performed on a material specimen—typically shaped like a cylindrical or rectangular bar—under load (pulling force) until the specimen cracks. The ultimate tensile strength is then determined by dividing the greatest load that the sample can withstand during the tensile test by the test specimen’s initial cross-sectional area.
- A material’s ultimate tensile strength can only be determined by knowing its cross-sectional area and the applied force. The formula (Stress (or) Strength = Force / Area) is used to determine a material’s ultimate tensile strength as well as the amount of stress it experiences. The main difference is that stress is an imposed load without failure, whereas strength is the load intended to fracture a material.
- Tensile strength is measured in pounds per square inch (PSI) in the imperial system, or force per unit area. Newtons per square meter, or N/m2, are employed in the metric system. Newtons per square meter, on the other hand, are used in place of Pascals (Pa); the names are the same.
- A material’s UTS can vary significantly depending on how it is treated and composed. For instance, high-grade steel, according to Industrial Metal Service, has a high UTS of over 1,000 megapascals (MPa), but aluminum alloys usually have a UTS of about 500 MPa.
- The materials used in applications like safety belts and suspension bridges need to be resistant to distortion. Appropriate materials can be selected for a given design by finding the material’s ultimate tensile stress.
- Engineers use tensile strength, particularly UTS, to determine whether a material is appropriate for a given application. It is a crucial factor to consider when choosing materials for equipment, buildings, and other components that will be exposed to force of any kind. For structures like bridges, buildings, or airplanes where maximum load and longevity are essential, materials with high UTS are typically chosen.
In general terms, ultimate tensile strength can be also described as:
- The ultimate tensile strength represents the peak point on the stress-strain curve, indicating the maximum stress a material can hold under tension before breaking.
- Often referred to simply as “tensile strength” or “the ultimate,” this value signifies the threshold at which a structure subjected to tension will fracture.
- Typically, the ultimate tensile strength exceeds the yield stress by a significant margin, sometimes by 50 to 60 percent, particularly in certain metals.
- When a ductile material reaches its ultimate strength, it undergoes necking, where the local cross-sectional area decreases. Notably, the stress-strain curve does not display any stress higher than the ultimate strength, although deformations may continue to increase. Following the attainment of ultimate strength, stress typically diminishes.
- Ultimate tensile strength is an intensive property, meaning its magnitude remains constant regardless of the specimen size. However, it is influenced by various factors including specimen preparation, the presence of surface defects, and the temperature of the testing environment and material.
- Ultimate tensile strengths span a wide range, from around 50 MPa for aluminum to as high as 3000 MPa for extremely high-strength steel.
How is Ultimate Tensile Strength Measured?
A tensile testing equipment, which holds the material in place with two clamps, is used to perform the ultimate tensile strength test. To apply the force, one clamp remains motionless while the other moves at a steady pace. The device tracks the material’s elongation and applied force until it breaks. Following the test, a graph is generated that displays the relationship between the force used and the material’s elongation.
Comprehensively, it is measured with following steps:
- Testing procedures carried out at material testing laboratories are used to ascertain a material’s tensile strength. In order to determine how much stress the specimen can sustain before breaking, various amounts of force are applied to it in different directions during the testing process.
- The material will be attached to a tensile machine by an engineer in order to get ready for the tensile strength testing procedure. These devices often have two or more points of attachment to the object. The specimen will then be continuously tensioned by the engineer using a tensile machine to pull and stretch it.
- Until the material breaks or deforms, engineers continuously watch the process and document changes to the material on a stress-strain curve. The force per cross-sectional area is the standard method for determining tensile strength. These exams are employed for a number of purposes, including:
- Selecting and identifying premium materials for production
- Estimating the materials’ performance for applications in real time
- Assessing a program’s adherence to quality standards, contracts, and specifications
- Testing new items to see if the material satisfies safety requirements
- Engineers evaluate product parts to make sure they satisfy standards, but they also look for specific information about the material they are testing. Tensile strength tests usually provide engineers with useful details about the characteristics of the material, including:
- Maximum load: The highest weight that a material is capable of supporting before failing or deteriorating.
- Ductility: The degree to which a material can be deformed before rupturing of any kind.
- Bend: The capacity of a substance to bend without breaking on its convex or concave sides.
- Elongation: A product’s elasticity and ductility under continuous weight-induced stress, which causes the material to lengthen.
- The information gathered from testing can be used to help manufacturers choose which products and materials to incorporate into their operations. These categories do not include tensile strength measurements. Rather, tensile strength is being measured in three separate methods by engineers during this testing:
- Yield strength: The maximum stress that a material can withstand before breaking is referred to as its yield strength. Additionally, when a material reaches its yield point, the specimen becomes permanently distorted and the alterations that happened during testing are irreversible.
- Ultimate strength: The maximum amount of stress that a material can withstand when being dragged or stretched is known as its ultimate strength. The highest load divided by the original cross-sectional area of the material yields the ultimate tensile strength formula. The ultimate tensile stress is also indicated by the ultimate strength.
- Breaking strength: The breaking strength of a specimen is the tension at which a material breaks because it can no longer withstand the stress.
The ultimate tensile strength test is significant because it offers insightful data regarding the behavior of the material under stress. It facilitates understanding of the material’s performance in practical applications by engineers and manufacturers, such as building, bridge, and aircraft construction. Prior to the material being utilized in a product, the test helps in identifying any flaws or weaknesses in the material.
Formula for Ultimate Tensile Strength
The ultimate tensile strength formula is: Strength = Force / Area
The cross-sectional area of the test sample before deformation is represented by the area, and the force is the load necessary to fracture the material. The equations for ultimate tensile stress and tensile strength are similar. On the other hand, strength is the force required to break the sample per area, whereas stress is the amount of force applied per area.
Factors Affecting Ultimate Tensile Strength
The composition, microstructure, and manufacturing process of a material are some of the variables that can impact its ultimate tensile strength. Metals with a lower carbon concentration are more ductile and can withstand greater elongation before breaking, whereas metals with a higher carbon content often have higher tensile strength. The tensile strength of a material can also be influenced by its microstructure, which includes the size and distribution of its grains. Furthermore, modifications made to the material during the production process, such as heat treatment or cold working, might change its characteristics and tensile strength.
Below are the major factors that affect tensile strength:
- Material Composition: One of the primary factors affecting a material’s ultimate tensile strength is its composition. A material can acquire variable degrees of strength from different elements and alloys. For example, adding carbon to iron boosts its tensile strength and creates steel, which has better mechanical qualities.
- Microstructure: A material’s internal structure, which includes its phase distribution, grain size, and grain boundaries, has a significant influence on its final tensile strength. Finer-grained materials tend to have higher tensile strengths because of the strengthening of their grain boundaries, which prevents deformation and dislocation movement.
- Heat Treatment: By changing a material’s microstructure, heat treatment procedures like quenching, tempering, and annealing can have a big impact on its final tensile strength. For instance, tempering can increase toughness without sacrificing strength, whereas quenching at high temperatures can result in a martensitic microstructure with higher strength.
- Imperfections and Defects: Imperfections in a material, such as voids, dislocations, or inclusions, can function as stress concentrators and lower the material’s ultimate tensile strength. Lower defect counts are generally associated with better tensile strengths.
- Strain Rate: A material’s ultimate tensile strength may be impacted by the rate at which it experiences tensile loading. While dynamic factors like strain rate sensitivity may result in a fall in strength in some materials, higher strain rates in others can contribute to strain rate hardening and enhanced strength.
- Alloying Elements: An alloying element can change a base material’s mechanical characteristics, such as its ultimate tensile strength. Chromium, nickel, and molybdenum are common alloying elements used to increase the strength and resistance to corrosion of metals such as stainless steel.
- Temperature: The ultimate tensile strength of a material is significantly influenced by temperature. Increased temperatures may encourage weakening mechanisms including creep, thermal softening, or grain growth. On the other hand, at higher temperatures, certain materials show better ductility, which influences their overall tensile performance.
- Testing Conditions: A material’s determined ultimate tensile strength can be affected by variables such the testing environment, loading arrangement, and specimen geometry. In order to guarantee accurate and repeatable results, standardized testing processes are necessary.
Examples of the Ultimate Tensile Stress of Various Materials
- Steel: Steel is a mixture of iron (99%) and carbon (1%), giving the basic iron metal more strength and hardness. The tensile strength of steels is between 330 and 480 MPa. Steel, one of the most widely used metals in the world, is primarily utilized for structural purposes in the building of civil engineering projects and automobile structural components.
- Aluminum: Because of its inexpensive cost and good strength-to-weight ratio, aluminum is also one of the most widely used metals. Because of these characteristics, aluminum finds extensive use in aircraft and aviation. The maximum strength that aluminum can reach is about 100 MPa.
- Titanium: Titanium is a silver metal that occurs naturally. It is the most expensive metal due to its tremendous strength-to-weight ratio, yet processing it is quite difficult. Moreover, titanium is biocompatible. This makes titanium suitable for usage in aerospace and medical fields. The tensile strength of titanium is 900 MPa.
- Brass: Brass is a zinc and copper alloy. Due to its high conductivity and malleability, brass may be easily molded into intricate profiles. Brass is appropriate for use in electrical and plumbing applications because of these qualities. The tensile strength of brass is around 250 MPa.
- Copper: Because of its superior conductivity and resistance to corrosion, copper is used in heat exchangers, plumbing, and electrical wiring even though metal is not as robust as titanium or steel. Copper usually has an ultimate tensile stress of 200 MPa to 300 MPa.
- Rubber: Elastomeric materials, both natural and synthetic, are utilized in tires, gaskets, and seals, among other applications. Rubber has a comparatively low ultimate tensile stress, usually between 10 and 30 MPa.
- Concrete: Concrete, a composite material made of cement, aggregates, and water, is widely used in construction for its compressive strength. However, its tensile strength is relatively low, typically ranging from 2 MPa to 5 MPa.
Application of Ultimate Tensile Strength
- Manufacturing Operations: For procedures like forming, machining, and welding, it is crucial to comprehend the UTS of the materials. In order to prevent flaws or component failure, manufacturers must make sure that their materials can sustain the stresses used in these operations without going above their UTS. For instance, engineers must select materials with UTS values suitable for the necessary deformation without resulting in fracture or severe strain hardening during metal forming operations like stamping or extrusion.
- Material Selection: When choosing the right material for a given application, engineers take the ultimate tensile strength into consideration. For instance, high UTS materials are favored in structural engineering for load-bearing elements like beams, columns, and bridges since these components must be strong and long-lasting. In contrast, applications requiring flexibility and deformation resistance, such car body panels or packing materials, may select materials with lower UTS but higher ductility.
- Quality Control: To ensure consistency and dependability in manufactured goods, UTS is an essential criterion in quality control processes. Manufacturers are able to confirm that their materials fulfill predetermined performance norms and standards by testing material samples for their UTS. Variations in material composition, processing circumstances, or quality faults that may need to be fixed to maintain product integrity can be indicated by deviations from expected UTS values.
- Product Design: To make sure that products fulfill performance and safety standards, designers make use of UTS data. For example, engineers build aircraft components utilizing materials with high UTS-to-weight ratios to increase strength while minimizing bulk, as is necessary in the aerospace industry where weight reduction. Similar to this, when designing consumer electronics, material with the right UTS values is chosen so that the mechanical stresses incurred during operation can be tolerated without failure.
- Safety and Regulatory Compliance: Following safety rules and guidelines is crucial in sectors including aerospace, automobile, and construction. UTS data is used to prove regulatory compliance and guarantee that structures and their constituent parts can sustain expected loads and environmental conditions without failing. For instance, in order to guarantee structural integrity and occupant safety, building rules may stipulate minimum UTS criteria for construction materials.
Advantages and Disadvantages of High Tensile Strength
Some of the advantages of high tensile strength are:
- Strengthened resistance to strong forces is one of the main benefits of having a high tensile strength, especially a high ultimate strength.
- Heavy-duty applications like building construction, automotive parts, and aviation components benefit greatly from the endurance and ability of materials with high ultimate strength to withstand considerable tensile stresses without breaking. These materials also frequently have a high resistance to wear and impact, which extends their lifespan and increases the dependability of the systems in which they are utilized.
Some of the disadvantages of high tensile strength are:
- High tensile strength frequently has a significant cost.
- Although these materials are excellent at withstanding large stresses, they can lack ductility and flexibility.
- Even though they have extremely high tensile strengths, brittle materials like ceramics and some alloys are less resilient to abrupt impacts and stress concentrations, which increases the likelihood of catastrophic failure when the applied force exceeds their UTS.
- Additionally, more complex and costly production procedures are frequently needed to produce materials with a high UTS, which could increase expenses.
References
- https://www.metalsupermarkets.com/what-is-ultimate-tensile-strength/
- https://www.xometry.com/resources/materials/ultimate-tensile-strength/
- https://www.nuclear-power.com/nuclear-engineering/materials-science/material-properties/strength/stress-strain-curve-stress-strain-diagram/ultimate-tensile-strength-uts/
- https://www.americanmicroinc.com/resources/ultimate-tensile-strength/