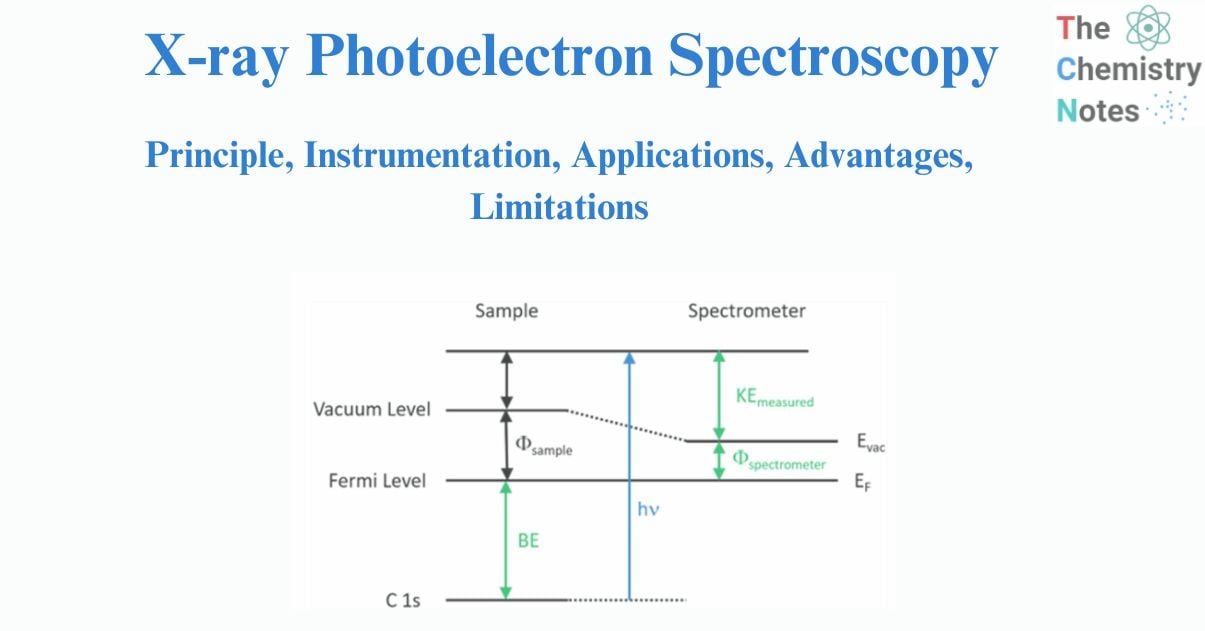
X-ray photoelectron spectroscopy is a surface-sensitive analytical technique that involves bombarding a material’s surface with X-rays and measuring the kinetic energy of the released electrons. The surface sensitivity and capacity to reveal chemical state information from the elements in the sample are two significant properties of this approach that make it powerful as an analytical tool. X-ray Photoelectron Spectroscopy (XPS), also known as Electron Spectroscopy for Chemical Analysis (ESCA), is a technique used to assess the quantitative atomic composition and chemistry of a substance. When a sample is exposed to monochromatic X-rays, photoelectrons with energy characteristic of the elements inside the sampling volume are emitted.
The number of electrons versus their binding energy is plotted to generate an XPS spectrum.
In the 1950s and 1960s, Kai Siegbahn (b. 1918) was working at Uppsala University in Sweden, where he invented modern-day XPS. Siegbahn was a physicist who named his method electron spectroscopy for chemical analysis (ESCA) because he recognized the value of the chemical information provided by XPS or ESCA. In 1981, he received the Nobel Prize in Physics for his contributions.
In X-ray photoelectron spectroscopy, Soft X-rays are used to ionize atoms and release core-level photoelectrons throughout the examination. Because the kinetic energy of escaping photoelectrons restricts the depth from which they can emerge, X-ray photoelectron spectroscopy has a high surface sensitivity and sampling depth of a few nanometers. The apparatus collects and analyses photoelectrons to generate a spectrum of emission intensity versus electron binding energy. As each element has a distinct set of binding energies, XPS can be used to identify the elements on the surface. Except for hydrogen and helium, all elements may be detected, and XPS has been used to analyze the surface of nearly every substance, from plastics to fabrics to soil to semiconductors.
Interesting Science Videos
Principle of X-ray photoelectron spectroscopy
Surface analysis by X-ray photoelectron spectroscopy involves the irradiation of the sample to low-energy (and mono-energetic) x-rays and then analyzing the energy of released electrons. Kα lines of Mg (1.2536 keV) and Al (1.4866 keV) are often used sources of X-rays. The penetrating depth of these photons in solids is restricted to a few microns. Thus, interactions between soft photons and surface atoms occur, resulting in the photoelectric emission of electrons. Core electrons with kinetic energy K.E are ejected by incident X-rays. The kinetic energy (K.E.) of the released electrons is calculated as follows:
K.E. = hν – B.E. – φs……………………… (1)
here hν is the energy of the photon, B.E. is the binding energy of the atomic orbital from which the electron is released, and φs is the work function of the spectrometer.
To determine the binding energy of an electron, Eq. (1) can be rearranged to obtain Eq. (2), where the terms on the right are either known (hν and Φspec) or measured in the XPS experiment (KE),
BE = hν – KE – φs…………………… (2)
The Binding Energy (BE) is characteristic of the core electrons for each element. XPS analysis is based on the idea that each element has a distinct collection of B.E. As a result, the concentration of components at the surface can be recognized and calculated. Any electron with a binding energy less than the x-ray source energy should be emitted from the material and seen using the XPS technique.
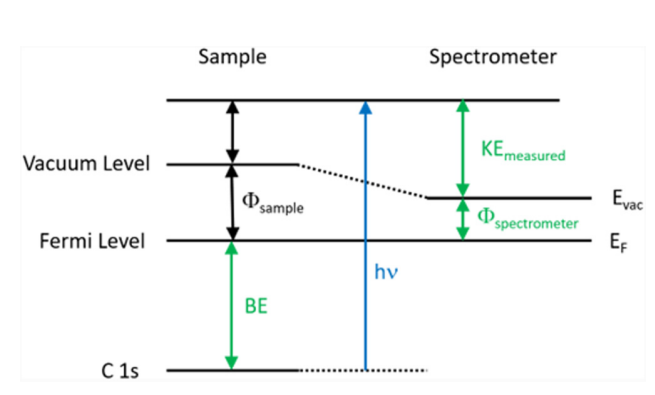
Fig: Energy level diagram illustrating the basic principle of XPS
The binding energy of an electron is the property of a material that is unaffected by the X-ray source used to expel it. Because of changes in the chemical potential and polarizability of compounds, the B.E. of elements varies (the chemical shifts). These changes allow the chemical condition of the object under investigation to be determined.
Instrumentation of X-ray photoelectron spectroscopy
Instrumentation of X-ray photoelectron spectroscopy contains an X-ray source, sample stage, extraction lenses, analyzer, and detector housed in an ultra-high vacuum environment.
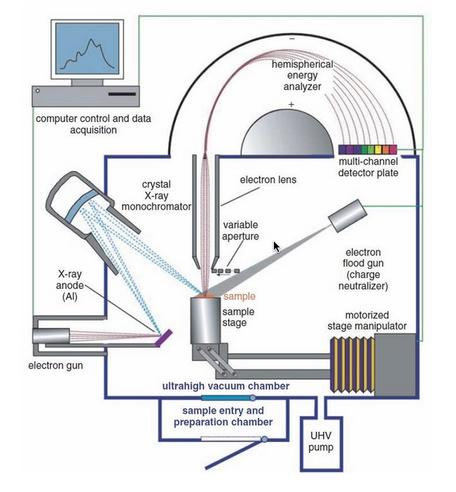
Fig: Instrumentation of X-ray photoelectron spectroscopy
Image source: https://serc.carleton.edu/msu_nanotech/methods/xps.html
Ultra-high vacuum environment
Because the radiated electrons must not scatter off air molecules while traveling to the analyzer, XPS equipment is housed in ultra-high vacuum (UHV) settings, with vacuum levels on the order of 10-5 -10-6 m bar. In practice, X-ray photoelectron spectroscopy systems often have significantly lower base pressures of
10-9 to 10-10 m bar. Furthermore, X-ray photoelectron spectroscopy instruments use the UHV environment to decrease surface contamination within the chamber.
So, the ultra-high vacuum environment of the XPS instrument facilitates the transmission of the ejected electrons from the sample surface to the analyzer and reduces surface contamination. Additionally, an ultra-high vacuum environment is used to:
- Remove the sample’s adsorbed gases
- Eliminate contaminant adsorption on the sample
- Prevent arcing and high voltage breakdown.
- Enhance the mean free path of electrons, ions, and photons.
X-ray source
In contrast to electron sources, the energy of an X-ray source is determined by the anode material used, resulting in the availability of a number of discrete energies rather than a continuous change in energy as with electron and ion guns.
The photon energy must be strong enough to trigger intense photoelectron peaks in all elements of the periodic table. It is critical to consider the energy resolution of the primary X-rays while performing XPS analysis.
In XPS, an anode coated in Al or Mg is bombarded by electrons at a high voltage of between 10 and 15 kV, which is typically utilized as the source of X-rays. In this, AlK and MgK radiation lines are created with energies of 1486.6 eV and 1256.6 eV, respectively. Photons with these energies have enough energy to excite the core-level electrons in all atoms.
Lens system
A collection of electron optics called extraction lenses is located between the sample and the analyzer and serves numerous functions. These lenses specify the acceptance angle for collecting electrons emitted by the sample. A large acceptance angle is typically employed to improve electron collecting efficiency, while there are some investigations that favor smaller acceptance angles (angle-resolved XPS). The electron lens gathers and slows photoelectrons released from the sample surface before they enter the X-ray photoelectron spectroscopy energy analyzer.
Energy analyzer
The most important characteristics of the analyzer are energy range, energy resolution, sensitivity, and acceptance angle. Normally, its functions include delaying the incoming electron, selecting electrons with the appropriate kinetic energy (pass energy), and detecting electrons. The analyzer must have the following characteristics:
- Accurate energy measurement over a wide energy range
- Capability for high-energy resolution
- The ability to specify and, if possible, adjust the analysis area
- High dynamic range and low noise detector
The cylindrical mirror analyzer (CMA) and the hemispherical sector analyzer (HSA) are the most common analyzers used in X-ray photoelectron spectroscopy. However, because of its great energy resolution, the concentric hemispherical analyzer is frequently preferred. Usually, the analyzer radius affects resolution, with larger radius analyzers offering better energy resolution.
Detector
A multichannel display detector, similar to a photographic plate, simultaneously detects the kinetic energy of the ejected electrons.
Recorder
The high-performance computer functions as a recorder/monitor, controlling the voltage to the lens and analyzer. It also generates an XPS spectrum. Computers automatically produce XPS spectra by plotting Ek intensity vs binding energies.
Applications of X-ray photoelectron spectroscopy
Qualitative analysis
As the core level binding energy is a property of an element or atom that produces an XPS spectrum, XPS provides information on the elemental composition of the material surface as well as the chemical bonding of the elements. The electrical structure of an atom is intimately related to the Xps peaks. Spectral peaks are indexed similarly to the electrical levels from which photoelectrons are emitted.
Quantitative analysis
Since the intensity of distinct electron transitions of a given element is directly proportional to the amount of that element present in the sample being studied, XPS provides a convenient method of quantitative chemical analysis. Specifically, the intensities of the XPS spectra depend on the atomic concentration of the sample’s considered element.
Chemical shift
XPS can identify the same element in diverse contexts. It is conceivable because the binding energy of an electron in an atom is sensitive to variations in charge density generated by chemical bond formation. Chemical shift refers to the difference in binding energy between the same atom in two chemically distinct places in the same compound or two different compounds.
The chemical shift is distinguished by the kind and number of atoms surrounding the sample’s ionized atom. It is caused by variations in the electrostatic screening of the core electrons when the valence electrons in the ionized atom are attracted or rejected. It can also be used to determine the type of bonding present in an oxidized sample. The chemical shift provides information about the chemical binding nature, the nature of functional groups, the different oxidation states of atoms or elements, and so on.
Thickness measurements
Xps is a useful tool for estimating the approximate thickness of a thin surface film less than 10 nm by comparing the intensity of the core level electron from an element within the film to the intensity of the core level electron from an element present in the thin surface film’s underlying substrate.
In-depth analysis
In-depth surface analysis is the estimation of the in-depth distribution of constituents in a thin surface coating. It is done to determine whether or not the thin layer is homogeneous. The escape depth of the produced photoelectrons is used in the in-depth analysis. The escape depth is determined by the electron’s inelastic mean free path. The average distance between successive inelastic collisions on the sample’s surface is defined as the inelastic mean free path. The electron is no longer useful for identifying the source atom after an inelastic collision since it has lost some of its kinetic energy. If this electron reaches the deterrent, it will be counted as a background in the spectrum
- XPS is also used for the evaluation of material processing steps like cleaning, plasma etching, oxidation, and thin film formation.
- In surface chemistry, it is used for the analysis of adhesion, corrosion, biocompatibility, lubrication, catalysis, surface cleanliness, processing residues, thin layer composition, migration of contaminants or additives from the bulk, and so on.
Advantages of X-ray photoelectron spectroscopy
- X-ray photoelectron spectroscopy is a non-destructive method.
- It has a high rate of resolution.
- It has a very high surface sensitivity (1-10 nm analysis depth).
- Surface chemical state identification
- Except for H and He, all elements may be identified.
- Quantitative analysis, including changes in chemical states between samples
- Suitable for a wide range of materials, including insulating samples (such as paper, plastics, ceramics, and glass).
- Depth profiling for significant elements (usually greater than one atomic percent) allows for the study of the catalyst surface before and after use.
Limitation of X-ray photoelectron spectroscopy
- Poor spatial resolution due to a lack of adequate spatial resolution
- Absolute sensitivity is only mild.
- XPS is able to analyze elements with atomic numbers of 3 or above, hence it cannot identify hydrogen or helium.
- XPS spectra are also time-consuming to obtain.
- It is not appropriate for trace analysis.
- An ultra-high vacuum is necessary to get an accurate result.
- X-ray photoelectron spectroscopy instrument is more expensive as compared to other surface analysis instruments.
- Detection limits are typically ~ 0.1 at%.
- The smallest analytical area is ~30 µm
- There is an absence of specific organic information (no long-range bonding information).
- Samples must be suitable for use in a high vacuum environment.
References
- http://site.iugaza.edu.ps/nashgar/files/2017/01/XPS.pdf
- https://chem.libretexts.org/Courses/Franklin_and_Marshall_College/Introduction_to_Materials_Characterization__CHM_412_Collaborative_Text/Spectroscopy/X-ray_Photoelectron_Spectroscopy_(XPS)
- https://serc.carleton.edu/msu_nanotech/methods/xps.html
- https://epgp.inflibnet.ac.in/epgpdata/uploads/epgp_content/S000831ME/P001676/M030247/ET/1525950662Module-5_Unit5_COM-I.pdf
- https://www.innovatechlabs.com/newsroom/2012/advantages-disadvantages-xps-testing-analysis/