3D printing, also known as additive manufacturing, transforms production by building three-dimensional products layer by layer from digital designs. 3D printing, an additive manufacturing technique, constructs objects by layering materials without the need for molds or large material blocks. It offers rapid production, low setup costs, and the ability to create complex geometries using various materials. This technology finds extensive application in engineering, especially for prototyping and fabricating lightweight structures. Industries like hearing aids, aviation, and automotive leverage 3D printing for prototyping and custom production, utilizing tailored scans to streamline manufacturing processes.
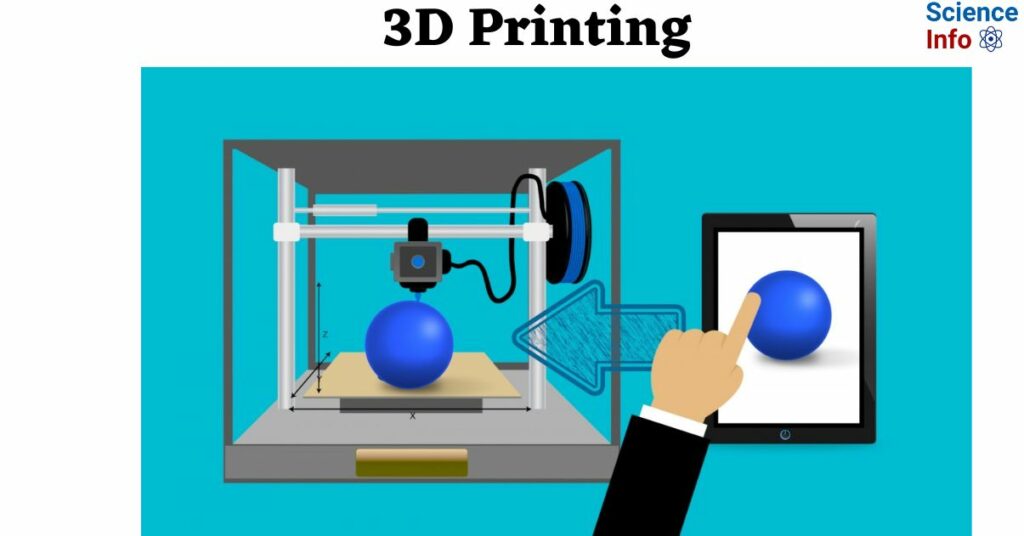
While current 3D printing speeds may not yet support mass production, they significantly reduce lead times for prototype development and tooling, benefiting small-scale manufacturers by cutting costs and accelerating time to market. The manufacturing, logistics, and inventory management sectors are likely to be affected by 3D printing as it develops further, possibly changing mass production procedures. By allowing for faster, more cost-effective prototyping and the development of detailed designs with minimal material waste, 3D printing promotes innovation and the growth of product-based businesses.
Interesting Science Videos
What is 3D Printing?
3D printing, also referred to as additive manufacturing, is a revolutionary process that transforms digital designs into physical objects by depositing material layer by layer. This innovative technology enables the creation of intricate shapes and structures with remarkable precision and efficiency.
Unlike traditional manufacturing methods that often involve subtracting material from a solid block, 3D printing builds objects from the ground up, adding material only where needed. This approach not only minimizes waste but also opens up possibilities for producing complex geometries that would be challenging or impossible to achieve through conventional means.
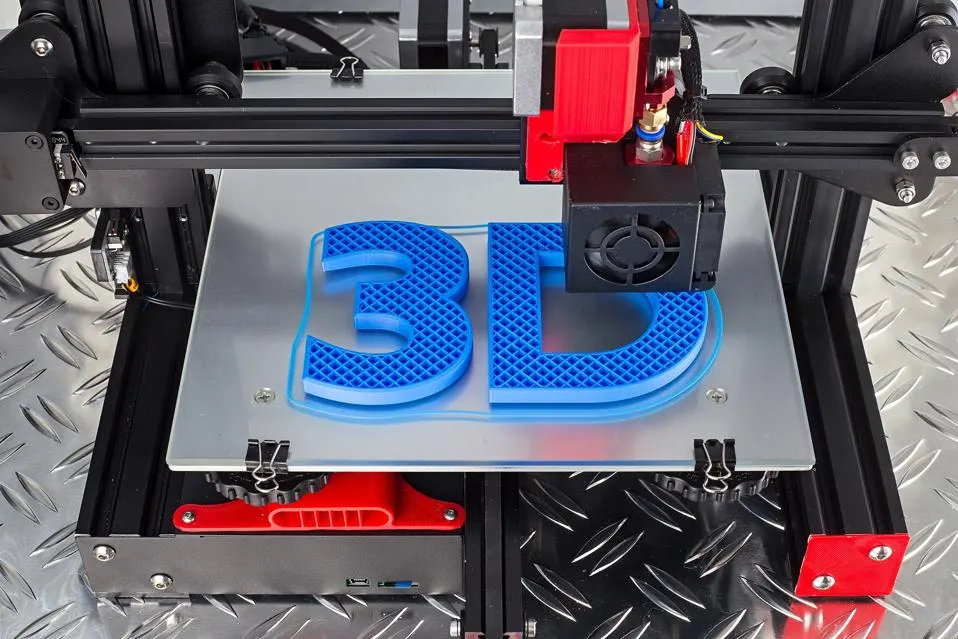
At the heart of 3D printing is a digital model, typically created using computer-aided design (CAD) software. This model serves as a blueprint for the printer, guiding its movements as it deposits successive layers of material to form the final object. Various materials can be used in 3D printing, including plastics, metals, ceramics, and even food-grade substances, depending on the requirements of the application.
One of the key advantages of 3D printing is its accessibility. With the right equipment and software, virtually anyone can harness the power of additive manufacturing to bring their ideas to life. This democratization of manufacturing has fueled innovation across a wide range of industries, from aerospace and automotive to healthcare and consumer goods. Moreover, 3D printing offers significant cost savings compared to traditional manufacturing methods, particularly for low-volume production runs or custom-designed products. By eliminating the need for specialized tooling and reducing material waste, additive manufacturing can streamline the production process and accelerate time to market.
In addition to its economic and practical benefits, 3D printing has also opened up new avenues for creativity and experimentation. Artists, designers, and engineers alike are pushing the boundaries of what’s possible with this versatile technology, exploring novel materials, intricate designs, and functional prototypes that were once unimaginable.
In conclusion, 3D printing represents a paradigm shift in manufacturing, enabling on-demand production of complex objects with unprecedented speed, flexibility, and affordability. As this transformative technology continues to evolve, its impact on industry, society, and creativity is poised to grow exponentially.
How does 3D Printing Work?
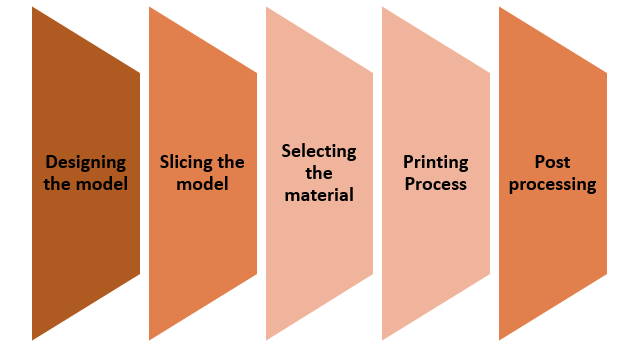
Designing the Model: Begin by creating a 3D model using computer-aided design (CAD) software or by scanning an existing object with a 3D scanner.
Slicing the Model: Slice the digital model into thin horizontal layers using slicing software. Each layer represents a cross-section of the object to be printed. The slicing software calculates the toolpath for the 3D printer.
Selecting the Material: Choose the appropriate material based on the application requirements. Materials commonly used in 3D printing include thermoplastics, metals, ceramics, resins, and composites. Each material has unique properties that affect the final characteristics of the printed object.
Printing Process: Various 3D printing technologies are available:
- Fused Deposition Modeling (FDM): Thermoplastic filament is melted and deposited layer by layer through a heated nozzle.
- Stereolithography (SLA): Liquid photopolymer resin is selectively solidified layer by layer using a UV laser.
- Selective Laser Sintering (SLS): High-powered laser selectively fuses powdered material layer by layer.
- Digital Light Processing (DLP): Liquid resin is cured layer by layer using a digital light projector.
Post-Processing: After printing, the object may require post-processing:
- Removal of support structures if needed.
- Sanding, polishing, or other surface treatments to improve the finish.
- Additional treatments such as painting or plating may also be applied.
Each step in the 3D printing workflow is essential for creating high-quality printed objects. From designing the model to selecting the material and printing process to post-processing, careful attention to detail ensures successful outcomes in 3D printing projects.
History of 3D Printing
Early Days in Rapid Prototyping (Late 1980s – Early 1990s):
- Additive manufacturing first found its footing in rapid prototyping (RP), allowing for quicker production of prototypes.
- RP enabled designers to use computer-aided design (CAD) software to create models, with machines following these digital blueprints to build objects layer by layer.
- This method of creating objects by “printing” their cross-sections became known as 3D printing.
Innovations at MIT and 3D Systems:
- In the early 1990s, Massachusetts Institute of Technology (MIT) developed a process known as 3-D Printing (3DP) and licensed it to companies for further development.
- 3D Systems, established in 1986, led the charge in developing various 3D printing methods and patented technologies like stereolithography apparatus (SLA) and selective laser sintering (SLS).
Technological Advancements:
- Over time, 3D printing technology evolved to not only create prototypes but also produce finished products.
- Improvements include finer detail printing, shorter post-printing cleanup, and the ability to use a wider range of materials, including metals and ceramics.
- 3D printers now come in various sizes, from industrial models resembling small cars to desktop versions the size of microwave ovens.
Comparison with CNC Machining:
- Additive manufacturing, or 3D printing, is often compared to computer numerically controlled (CNC) machining, which removes material to create objects.
- Unlike CNC machining’s subtractive process, 3D printing builds objects layer by layer using materials like plastics, metals, and ceramics.
Types of 3D Printers
Stereolithography (SLA)
- SLA printers utilize a UV laser to cure liquid resin into solid plastic.
This process excels in producing highly detailed parts with smooth surfaces and tight tolerances. - Widely used in industries such as engineering, product design, and dentistry due to its accuracy and fine details.
- Common applications include anatomical models and microfluidics in the medical field.
- Although parts may require support structures during printing, these can be removed post-printing.
- SLA is favored for rapid prototyping and can also be used for low-volume production of complex geometries.
Selective Laser Sintering (SLS)
- SLS printers fuse nylon-based powders into solid plastic using a high-power laser.
- Parts made through SLS are durable and exhibit excellent mechanical properties, suitable for functional testing.
- Supports are unnecessary in SLS, allowing for efficient use of the build platform and nesting of multiple parts.
- Often used for prototyping designs intended for injection molding due to its ability to simulate final part properties.
- Ideal for producing parts with complex geometries and features like living hinges and snap-fits.
Fused Deposition Modeling (FDM)
- Also known as Fused Filament Fabrication (FFF), FDM printers extrude thermoplastic filaments through a heated nozzle onto a build platform.
- Widely used in consumer-level 3D printing due to its affordability and accessibility.
- Suitable for basic prototyping and low-cost production of simple parts, though with lower resolution compared to SLA or SLS.
- Industrial FDM printers offer soluble supports and a wider range of engineering thermoplastics, expanding its utility.
Digital Light Processing (DLP)
- Similar to SLA, DLP cures liquid resin using light, but it employs a digital light projector screen instead of a UV laser.
- Offers faster build speeds compared to SLA due to imaging entire layers at once.
- Frequently used for rapid prototyping and low-volume production runs of plastic parts.
- Suitable for applications requiring high throughput and moderate surface finish requirements.
Multi Jet Fusion (MJF)
- Utilizes an inkjet array to apply fusing agents to a bed of nylon powder, followed by heating to fuse each layer.
- Offers consistent mechanical properties and an improved surface finish compared to SLS.
- Accelerated build times lead to lower production costs, making it suitable for production-level quantities.
- Commonly used for functional parts with complex geometries in various industries.
PolyJet
- Enables fabrication of parts with multiple properties such as colors and materials.
- Suitable for prototyping elastomeric or over-molded parts, saving costs on tooling during the development cycle.
- Offers versatility in material selection and can simulate a wide range of final part properties.
- Recommended for designs requiring multi-material or multi-color capabilities, where SLA or SLS may be less economical.
Direct Metal Laser Sintering (DMLS)
- Used for 3D printing metal parts by sintering metal powder using a laser.
- Ideal for complex geometries and lightweight parts with internal channels or hollowed-out features.
- Offers dense metal parts comparable to those produced through traditional manufacturing methods like machining or casting.
- Suitable for both prototyping and production applications, especially in industries like aerospace and medical.
Electron Beam Melting (EBM)
- Another metal 3D printing technology uses an electron beam to melt metal powder in vacuum conditions.
- Offers high precision and is suitable for producing parts with complex geometries.
- Used for prototyping and production of metal components, particularly in industries requiring organic-like structures or intricate designs.
Each type of 3D printing technology has its unique advantages and limitations, making it crucial to select the appropriate process based on factors such as budget, mechanical requirements, cosmetic appearance, material selection, and geometry of the desired parts. Understanding these considerations allows for optimal utilization of 3D printing technology throughout the product development cycle, ultimately leading to better products.
3D Printing Materials
Over the years, the landscape of 3D printing materials has undergone significant evolution, offering a wide array of options catering to diverse applications and printing technologies.
Plastics: Plastics remain the cornerstone of 3D printing materials, offering versatility and reliability across various printing methods.
Nylon (Polyamide): Nylon, commonly used in both powder and filament forms, showcases strength, flexibility, and durability. Its adaptability in sintering processes or FDM printing makes it a preferred choice. Additionally, it can be colored pre or post-printing, widening its applications. The combination of nylon with powdered aluminum yields Alumide, a prevalent material for sintering.
ABS (Acrylonitrile Butadiene Styrene): ABS, prevalent in entry-level FDM printers in filament form, boasts exceptional strength and comes in an extensive range of colors. Its availability from non-proprietary sources further contributes to its popularity.
PLA (Polylactic Acid): PLA, a biodegradable plastic, has gained traction in 3D printing due to its eco-friendliness. Available in resin format for DLP/SL processes and filament form for FDM, PLA offers various color options, including transparency. However, it tends to be less durable and flexible compared to ABS.
LayWood (Wood/Polymer Composite): LayWood, designed for entry-level extrusion 3D printers, comes in filament form and constitutes a wood/polymer composite (WPC). This innovative material provides a unique aesthetic appeal akin to wood, expanding creative possibilities for 3D printing enthusiasts.
Application of 3D Printing
Medical and Dental: The medical field has embraced 3D printing for its ability to customize products, from prototypes for medical devices to dental crowns produced through metal casting. This technology facilitates the creation of personalized aids like hearing aids and orthotic insoles, enhancing patient care and surgical procedures.
Aerospace: Aerospace companies have integrated 3D printing into product development and prototyping, pushing boundaries in manufacturing. With rigorous standards and demanding research, industrial-grade 3D printing systems have become essential for producing non-critical aircraft components.
Automotive: Initially used for prototyping, 3D printing in the automotive industry has evolved to include manufacturing operations. Companies explore on-demand production of spare parts, reducing inventory costs and enhancing after-sales services.
Jewellery: The jewellery sector has undergone significant transformation with 3D printing, enabling new design freedoms and streamlining production processes. Designers can now create intricate pieces directly through 3D printing, speeding up production and reducing traditional steps.
Art / Design / Sculpture: Artists and sculptors leverage 3D printing for innovative expression and precision. Through 3D modeling and scanning, artists reproduce ancient sculptures for study, enriching artistic endeavors.
Architecture: 3D printing plays a vital role in architectural model production, offering architects an efficient method to showcase designs. Ongoing research explores 3D printing as a direct construction method, revolutionizing architectural practices.
Fashion: Fashion designers embrace 3D printing for its design flexibility and customization options. From accessories to haute couture, 3D printing inspires creativity and innovation in the fashion industry.
Food: While in early stages, 3D printing in the food industry promises new avenues for food preparation and presentation. From chocolate confections to experimental “meat” printing, the technology holds potential for culinary innovation.
Consumer Products: Consumer 3D printing is an emerging field, with efforts to make the technology more user-friendly. DIY kits, online services, and specialized applications pave the way for broader consumer adoption in the future.
3D Printing Softwares
The journey of 3D printing begins with software, encompassing a variety of programs tailored to different stages of the design and printing process. These software solutions range from 3D modeling tools to print simulations and slicer programs.
Choosing the Right Software: When selecting software for 3D printing, it’s crucial to consider the two primary methods of 3D modeling: solid modeling and surface modeling. Solid modeling involves creating virtual objects by defining and joining 3D shapes, often with predefined structures to which refined surface details are added later. On the other hand, surface modeling starts with 2D surfaces and shapes, which are then manipulated in a ‘freeform’ manner to craft 3D shapes.
Solid vs. Surface Modeling: While both solid and surface modeling can achieve similar outcomes, they excel in different scenarios. Solid modeling is preferred for its efficiency in creating simple and non-organic shapes, whereas surface modeling is more suitable for crafting complex and organic shapes at a faster pace.
Popular Software: Among professionals, software like SolidWorks, Fusion 360, and Rhino 3D stands out as the most widely used for their robust features and capabilities. However, there are also numerous free software options available for amateurs looking to explore 3D printing.
In addition to modeling software, there are other valuable tools in the realm of 3D printing software. Print simulation tools aid in visualizing and optimizing the printing process, while file error fixers help identify and rectify any issues in 3D models before printing, ensuring smoother printing experiences and higher-quality outputs.
Advantages of 3D Printing
- Accessibility: Previously limited to experts, 3D printing is now accessible in various settings such as homes, businesses, hospitals, and schools.
- Affordable Pricing: Cost-effective 3D printing promotes wider adoption of the technology, allowing more users to benefit from its capabilities.
- Customization: 3D printing offers limitless possibilities for printing objects of various shapes and sizes, customizable to meet specific needs.
- Constant Prototyping/Increased Productivity: Rapid prototyping capabilities enable designers to iterate and improve prototypes quickly, boosting efficiency and productivity.
- Improved Life Quality & Welfare: The technology enhances quality of life by facilitating the creation of essential parts and models for education, healthcare, automotive, and other sectors.
- Eco-Friendly: Compared to traditional manufacturing methods, 3D printing generates less waste, making it an environmentally friendly option.
Disadvantages of 3D Printing
- Violation of Copyrights: The ease of reproducing objects through 3D printing raises ethical concerns, as it can lead to copyright infringement if copyrighted objects are printed without authorization.
- Harm to Authenticity: Counterfeiting and piracy may increase with widespread 3D printing, damaging the authenticity and market demand for original products.
- Printing Weapons: 3D printing enables the production of potentially dangerous objects like firearms and knives, posing security risks as they can be easily concealed and undetected in public places.
- Scanning & Fraud: 3D printers can be misused to scan and replicate sensitive items such as identification cards, credit cards, and keys, facilitating fraud and identity theft.
References
- https://www.protolabs.com/resources/blog/types-of-3d-printing/
- https://formlabs.com/asia/3d-printers/
- https://3dprintingindustry.com/wp-content/uploads/2014/07/3D-Printing-Guide.pdf
- https://computer.howstuffworks.com/3-d-printing.htm
- https://www.xometry.com/resources/3d-printing/3d-printing-guide/