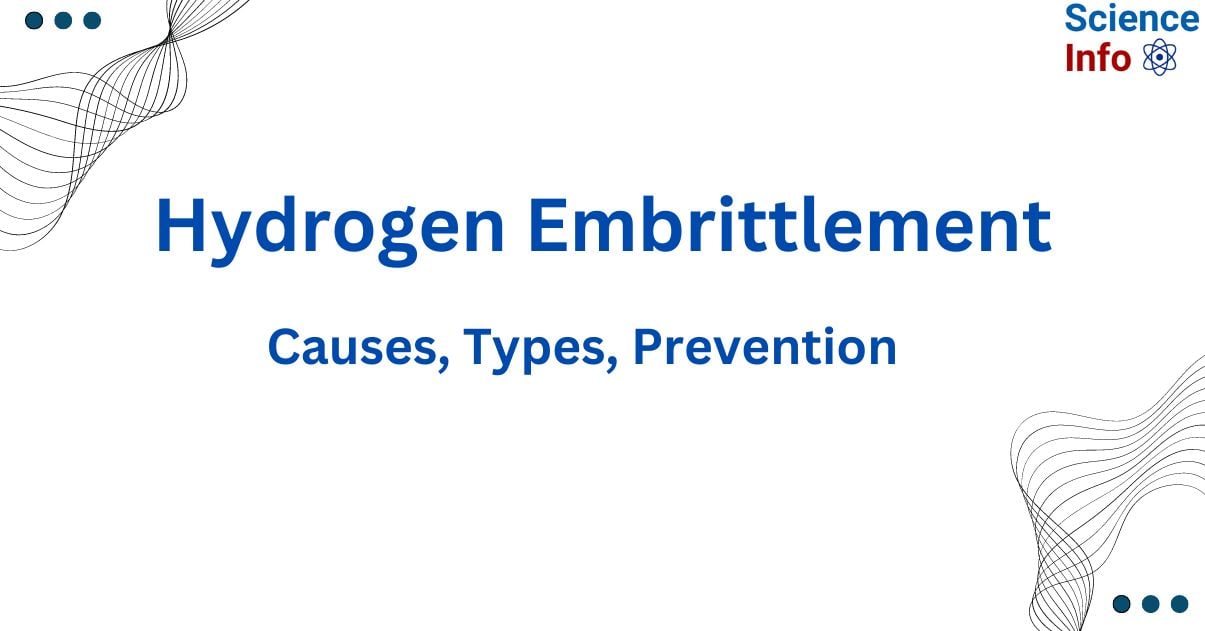
Hydrogen embrittlement is a loss of ductility caused by excessive hydrogen absorption, rendering the material brittle. This is because hydrogen atoms are substantially smaller than those that make up the deposited metal. This causes hydrogen atoms to migrate into the base metal’s crystal lattice and become trapped between the individual metal atoms. This can dramatically raise the stress of applied forces within the base metal, resulting in fracturing.
Hydrogen embrittlement is a phenomenon caused by the introduction of hydrogen into metal materials that results in reduced flexibility, and damage under low stress.
It takes place when hydrogen is introduced and diffused into metals, causing them to become brittle. Both the material’s microstructure and the quantity of hydrogen absorbed have an impact on the degree of embrittlement.
Interesting Science Videos
Types of hydrogen embrittlement
Several forces must work together to establish a condition in which hydrogen embrittlement occurs. A sensitive material comes first. Second, exposure to hydrogen. Finally, apply stress on the component. Failure due to hydrogen embrittlement cannot occur in the absence of all three.
Internal Hydrogen Embrittlement
Atomic hydrogen that enters the molten metal during the production process causes molecular imperfections as the metal solidifies.
External Hydrogen Embrittlement
It is caused by environmental factors such as intense heat, chemical treatments, electroplating, corrosion, cathodic protection, and operating in high-pressure hydrogen environments.
Causes of hydrogen embrittlement
External Loading or Residual Stress
Stress that exceeds a metal’s capability affects structural integrity, resulting in blisters, cracks, hydride development, and reduced flexibility or ductility.
Hydrogen Assisted Cracking (HAC) or Hydrogen Stress Cracking (HSC)
HAC or HSC occurs when atomic hydrogen reacts with the metal, causing fractures and material fractures.
Stress Corrosion Cracking (SCC)
Anodically polarised cracks and crevices promote more active corrosion, hastening structural degradation.
Mechanism of hydrogen embrittlement
Steel can absorb hydrogen atoms even at ambient temperature. Once absorbed, the atoms unite to form hydrogen molecules. As time passes, these molecules disperse through the metal, forming bubbles at grain boundaries. The bubbles exert pressure on the metal, lowering its ductility and tensile strength.
Normally, hydrogen can only enter metals as atoms or hydrogen ions. Therefore, gaseous hydrogen is not absorbed by metals at room temperature because it is in molecular form, where pairs of atoms are tightly bonded together. However, as the temperature rises, the molecules tend to split into single atoms, permitting absorption.
Molten material has higher rates of absorption, therefore casting and welding activities can present unique chances for hydrogen to enter metallic materials. Hydrogen ions are also created during reactions involved with corrosion, electroplating, and cathodic protection. As a result, hydrogen can easily enter metallic components.
Hydrogen embrittlement of a material can occur by many mechanisms, including hydride formation, hydrogen-enhanced decohesion (HEDE), hydrogen-enhanced local plasticity (HELP), and adsorption-induced dislocation emission (AIDE).
Hydrogen Enhanced Decohesion Mechanism (HEDE)
Troiano invented this mechanism in 1959. The process involves reducing a material’s cohesive strength at the fracture tip by introducing a hydrogen atom. When hydrogen atoms diffuse into a material due to stress, it reduces the interatomic strength and cohesive strength at the crack tip, leading to cleavage-like fractures. Reducing a material’s cohesive strength reduces surface energy and fracture stress, resulting in fractures below acceptable values.
Hydrogen-Enhanced Local Plasticity Model (HELP)
This mechanism, first stated in 1972, is now universally recognized. This model suggests that hydrogen accumulation near the fracture tip reduces the resistance to dislocation motion, resulting in increased mobility and plastic deformation in the metal lattice. Fracture modes such as intergranular, transgranular, and quasi-cleavage may occur depending on hydrogen content, microstructure, and stress intensity near the crack tip.
HELP is a well-known phenomenon in subcritical crack growth caused by dislocation motion at the crack tip and the accumulation of hydrogen atoms. The presence of hydrogen atoms caused local plastic distortion, resulting in brittle plastic flow. Hydrogen atoms collected at dislocation cores reduced elastic interaction between moving dislocations, causing dislocations to occur more easily. Because of this, applied stress for dislocation motion is reduced, flexibility is increased, and fracture formation occurs more readily at lower applied stress levels.
Adsorption-induced dislocation emission (AIDE)
This mechanism combines both HEDE and HELP. This method involves the adsorption of solute hydrogen atoms at stress-prone surfaces, such as crack points. Hydrogen adsorption at a crack tip weakens the material’s interatomic bonds and allows for dislocation injection, leading to crack propagation, slide, and microvoid formation via the HEDE and HELP mechanisms.
Hydrogen-Enhanced Macroscopic Ductility (HEMP)
It is also known as the hydrogen-enhanced macroscopic plasticity model. Hydrogen has a significant impact on the mechanical properties of steel. When a large volume (concentration) of the material interacts with hydrogen, it softens the solid solution and reduces the yield strength. Yielding caused plasticization throughout the specimen, resulting in macroscopic plasticity enrichment.
Why it is destructive?
Many businesses rely on steel structures, which can come into contact with hydrogen throughout operation or manufacture. These consist of oil and gas structures, wind turbine steel components, architectural buildings, and, of course, hydrogen storage and transportation infrastructure.
When hydrogen degrades the steel’s quality, these buildings must be replaced or repaired quickly, and there is a possibility of collapse over time. The oil and gas industry also faces a second threat: leakage. There is a chance of fire and explosion when significant amounts of hydrogen escape from an infrastructure.
Prevention of hydrogen embrittlement
Load Bearing ability: To prevent imbalances that could result in hydrogen stress cracking, carefully evaluate the fasteners’ load-bearing ability.
Material hardness: Pay particular attention to the material’s hardness to better understand its structure and capabilities.
Manufacturing Process: Take precise care during manufacture to limit the exposure of molten metal to atomic hydrogen.
Heat Treatment: Use heat treatment or baking procedures to remove any remaining atomic hydrogen in the material.
Another preventive process involves:
Cadmium and titanium plating
The plating procedure was originally established in 1960.
Initially, a cadmium cyanide solution was mixed with the titanium compound, which is soluble in cyanide. If the operation was carried out correctly, the titanium content of the deposit was between 0.1 and 0.5%. This process has been utilized to coat threaded rods, gear actuation cylinders, and related shafts that operate in high-stress environments.
Titanium was present in the non-cyanide solution at a concentration of 0.1-0.7%.
Titanium compounds are stable in non-cyanide solutions. Non-cyanide solutions are more effective than cyanide solutions for applying protective coatings to high-quality instrumentation steel, structural steel, and spring wire.
Iron plating
Ion plating, also known as physical vapor deposition, reduces hydrogen embrittlement problems. Since this process takes place in a vacuum, the likelihood of embrittlement is lowered.
Post-plating baking
Baking the item immediately after plating can typically counteract the effects of hydrogen embrittlement. Baking the component for four hours at 375º F within one hour of plating is recommended. Steels often require a lower temperature of 200-300ºF.
Substituting metal alloys
Some metals, notably exceptionally high-strength steels, are more prone to hydrogen embrittlement. Combining these high-risk metals with those that have lower hydrogen diffusion rates, such as nickel, zinc, or molybdenum, can be particularly efficient in preventing hydrogen embrittlement.
Adding inhibitors while pickling
Pickling may produce base metal corrosion, which could result in rapid hydrogen evolution and embrittlement. The application of inhibitors during the pickling stage may decrease or prevent this corrosion, resulting in a lower hydrogen pick-up.
References
- https://www.sciencedirect.com/topics/engineering/hydrogen-embrittlement#:~:text=Hydrogen%20embrittlement%20(HE)%20is%20the,corrosion%20resistance%20at%20elevated%20temperature.
- https://link.springer.com/referenceworkentry/10.1007/978-981-19-0740-1_235-1
- https://demaco-cryogenics.com/blog/hydrogen-embrittlement/
- https://www.penflex.com/hydrogen-embrittlement-causes-prevention/
- https://ntrs.nasa.gov/api/citations/19670021605/downloads/19670021605.pdf