The lanthanoid series comprises 14 elements, ranging from cerium to lutetium, and is commonly referred to as f-block metals due to the presence of 4f orbitals in their valence shell. These elements, named after lanthanum (La), which is itself a d-block metal, exhibit very similar chemical behavior. Consequently, lanthanum is often included in the lanthanoid series. The collective term “rare earth metals” encompasses the lanthanoid series along with lanthanum (La), scandium (Sc), and yttrium (Y).
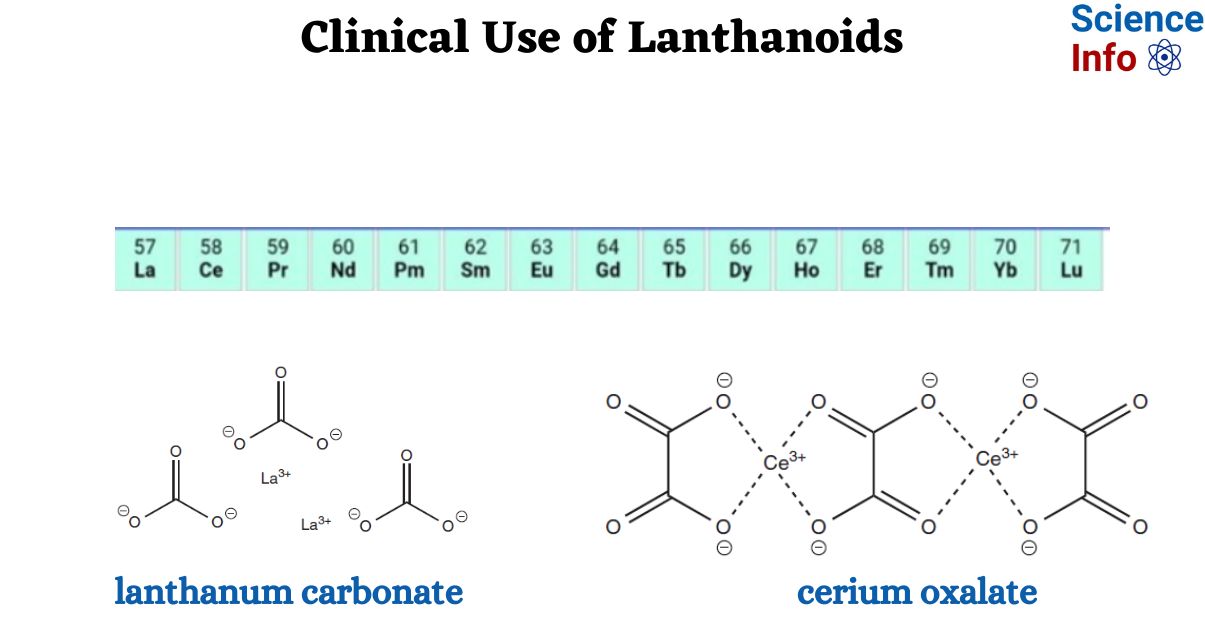
The lanthanoid series is often given the symbol Ln and referred to as the elements La–Lu.
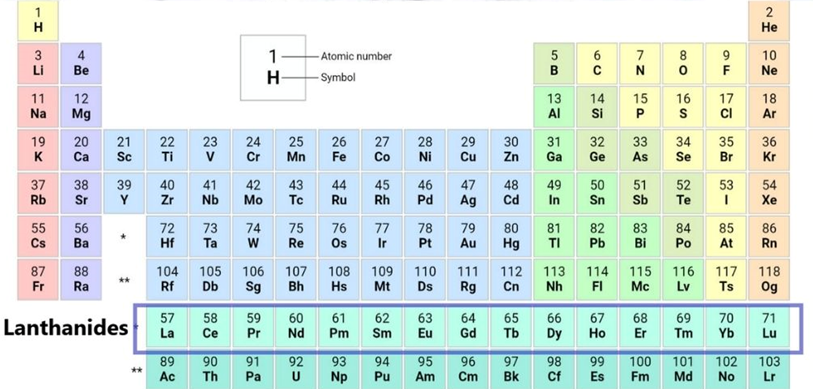
Interesting Science Videos
Lanthanoid series electronic configuration
Element name | Symbol | Ground-state electronic configuration |
Lanthanum | La | [Xe]6s25d1 |
Cerium | Ce | [Xe]4f16s25d1 |
Praseodymium | Pr | [Xe]4f36s2 |
Neodymium | Nd | [Xe]4f46s2 |
Promethium | Pm | [Xe]4f56s2 |
Samarium | Sm | [Xe]4f66s2 |
Europium | Eu | [Xe]4f76s2 |
Gadolinium | Gd | [Xe]4f16s25d1 |
Terbium | Tb | [Xe]4f96s2 |
Dysprosium | Dy | [Xe]4f106s2 |
Holmium | Ho | [Xe]4f116s2 |
Erbium | Er | [Xe]4f126s2 |
Thulium | Tm | [Xe]4f136s2 |
Ytterbium | Yb | [Xe]4f146s2 |
Lutetium | Lu | [Xe]4f146s25d1 |
Due to the lanthanoids‘ tendency to lose three electrons (6s2 and 5d1) due to their electrical structure, the trivalent cation is the most stable oxidation state. With different quantities of 4f electrons added, the resulting trivalent cations have an electrical configuration similar to that of xenon (Xe). Closer to the nucleus are the 4f electrons, which are shielded from it by the complete subshells 5s2 and 5p6. Triple-positive charged noble gases are another term frequently used to describe trivalent lanthanoids.
Biology and Toxicology of Lanthanoids
- Lanthanoids are mostly utilized in the manufacture of lasers, batteries, and other electronic equipment.
- A growing number of lanthanoid salts are being employed in clinical settings, such as phosphate binders for the treatment of renal osteodystrophy or as contrast agents (CAs) for magnetic resonance imaging (MRI). Examples of these salts are lanthanum, cerium, and gadolinium.
- Because of their identical ionic radii, lanthanoids (Ln) exhibit biological behavior that is almost identical to that of Ca2+.
- Since lanthanoids are primarily trivalent, they are more charged than Ca2+. Lanthanoids bind to water molecules and calcium-binding sites in biological molecules with a high affinity. Lanthanoids have a coordination number that ranges from 6 to 12. Typically, the lanthanoid ion is coupled with eight or nine water molecules. Compared to calcium, which has a coordination number of 6, this is a far lower value.
- Lanthanoid ions are known to inhibit the receptor-operated calcium channels in the human body. Even though lanthanoids are unable to pass through cell membranes, they nevertheless prevent the exchange of Na+/Ca2+ across synaptic plasma membranes, which prevents muscular contraction (e.g. in skeletal muscle or cardiac muscle). Additionally, lanthanoids can replace calcium in proteins and enzymes, which can either activate or inhibit the catalytic activity of those molecules. Because lanthanoids generally exhibit biological behavior similar to that of calcium ions, they can be utilized to investigate the mechanism of action of calcium ions in a range of biological contexts.
Examining the toxicity of lanthanoids is fascinating. Since lanthanoids are unable to pass through cell membranes and are consequently not absorbed when taken orally, they are generally not recognized as harmful. When given intravenously, lanthanoids can interact with a wide range of biological targets, making them poisonous. Acute poisoning manifests as a sharp drop in blood pressure and abrupt cardiovascular problems. Chronic poisoning might cause oedema and liver damage. Lanthanoids are frequently swiftly transported to the liver and bones following intravenous injection.
Clinical use of lanthanum carbonate
- The atomic number of lanthanum is 57, and its symbol is La. The metal is silvery white and signifies the beginning of the lanthanoid series (Ln). There are two oxidation states for lanthanum: +II and +III, with the latter being the more stable. The resultant La3+ ion has the electrical structure [Xe]4f0. When lanthanum burns in the presence of air, lanthanum(III) oxide is produced.
4La + 3O2 → 2La2O3
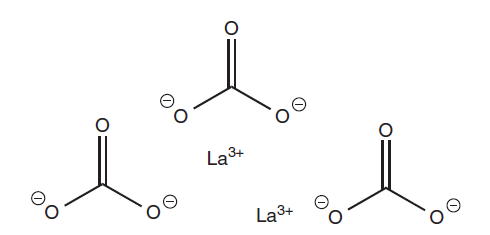
Chemical structure of lanthanum carbonate
- Due to the electropositive nature of lanthanum, it interacts with water to generate lanthanum hydroxide. Additionally, it generates the corresponding lanthanum halide salt when it combines with halogens.
- It is employed in the treatment of hyperphosphatemia, a condition characterized by elevated phosphate concentrations in the serum.
- Lanthanum carbonate has been effectively explored as a phosphate-binding agent, meeting the specified criteria for an efficient phosphate binder: it is non-toxic, rapidly binds phosphate, is not absorbed in the gastrointestinal tract, and is easily excreted.
- Comparative analysis of various lanthanum salts revealed that La2CO3⋅4H2O exhibits superior phosphate-binding properties across a range of pH levels. This implies that phosphate can be bound in the stomach (where pH is very low), and the complex remains stable during transit through the gastrointestinal tract, where pH is higher.
- Pharmacological investigations indicate that orally administered lanthanum carbonate is poorly absorbed, with over 90% excreted through feces. No specific toxicity has been observed.
- Lanthanum carbonate hydrate, marketed as Fosrenol, has obtained approval in Europe and the United States for clinical use in patients with chronic renal failure.
- Typically, 0.75–2.25 g of elemental lanthanum is administered as an initial dosage of lanthanum carbonate hydrate in divided doses with meals. Every two to three weeks, the dosage must be checked to reach a maintenance dose of 1.5 to 3 g. It is advisable to counsel patients to chew their medications before ingesting them. Constipation and diarrhea are the most frequent side effects caused by GI tract disruptions.
Clinical application of cerium salts
- The atomic number of cerium is 58 and its chemical symbol is Ce. It is a delicate, silvery metal that readily oxidizes in air. Three oxidation states exist for cerium: +II, +III, and +IV, with the latter being the more stable. The resultant Ce4+ ion has an electrical configuration of [Xe]4f0. The electrical structure that results from the rarest oxidation state, Ce+2, is [Xe]4f2.
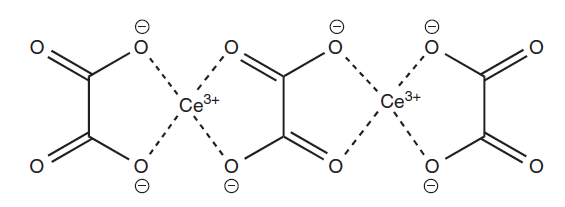
Chemical structure of cerium oxalate
- Since cerium (III) oxalate was first employed as an antiemetic in the middle of the 1800s, cerium has been used in clinical settings. Although the precise mode of action is unclear, the GI tract is thought to be the only area of localized impact. This is because cerium oxalate has poor water solubility and lanthanoids are not readily absorbed after oral treatment. However, antihistamines soon took the position of cerium salts in clinical settings as an antiemetic.
- Several Ce3+ salts were being studied for their antibacterial properties in burn wounds around the end of the 1800s.
- In particular, cerium nitrate [Ce(NO3)3] was utilized in conjunction with silver sulfadiazine because it demonstrated extensive action against a range of infections.
- The preliminary research yielded very positive results, with a 50% reduction in the death rate projected. This outcome was thought to be the result of both reagents’ synergistic antibacterial action.
- Cerium nitrate does not significantly affect microorganisms from burn wounds, according to recent studies.
- Moreover, studies have demonstrated that a major contributing factor to death in individuals with severe burn injuries is immune system suppression.
- Cerium nitrate has also been demonstrated to modulate the immune response linked to burns in animal models. These days, it’s thought that cerium(III) nitrate’s primary function when applied to burn patients in combination therapy.
- Currently, life-threatening burn wounds are treated with cerium salt in certain situations along with silver sulfadiazine.
- According to reports, there is an improvement in wound healing, a decline in mortality, and a considerable decrease in graft rejection rates.
Use of gadolinium salts as MRI contrast agents
- The atomic number of gadolinium is 64 and its chemical symbol is Gd.
- It is a ductile, silvery-white metal that is malleable. Gadolinium salts are primarily in the +III oxidation state, which results in the electronic configuration [Xe]4f7.
- When exposed to dry air, gallium is a reasonably stable metal. However, because it reacts slowly with water, it tends to oxidize when exposed to moisture.
- Gadolinium is employed in computer memory and compact disc production, as well as in microwave applications.
- In alloys, gadolinium is frequently utilized. Iron and chromium exhibit improved characteristics and workability with as little as 1% gadolinium.
- As a clinical use, gadolinium salt-containing solutions are utilized as CAs for MRI. This technique allows for the detection and observation of pathological and physiological changes in living tissue. The so-called relaxation properties of excited hydrogen nuclei present in human tissue’s lipids and water are what allow MRI to be used in medical applications. The term “relaxation” describes a phenomenon that is well-known in physics and chemistry, wherein a system takes longer to respond to an external stressor than it does to apply it. Excited hydrogen nuclei exhibit varying behaviors based on their surroundings in an MRI scanner’s strong magnetic environment.
- Although MRIs can be performed without the use of a CA, doing so can improve the imaging quality. The basic notion is that when a CA is present, the water relaxation rates are changed, resulting in more and/or enhanced information being displayed in the images. The CAs themselves are not visible in the photographs, in contrast to their function in X-ray imaging. These days, CAs are used for around one-third of MRI scans, and they allow for the detection of organ perfusions, renal clearance, and alterations in the blood–brain barrier.
- The trivalent cation Gd3+ serves as an effective contrast agent (CA) for magnetic resonance imaging (MRI) due to its paramagnetic nature. With the electronic configuration [Xe]4f7, Gd3+ possesses seven unpaired electrons in its valence shell, giving rise to its paramagnetic properties.
- The impact of Gd3+-based contrast agents on MRI image quality can be explained in two ways:
- (i) Gd3+ complexes with paramagnetic properties can coordinate water molecules and replace those in their surroundings, originally bound to the metal center. A typical Gd3+ complex features an eight-coordinated metal complex with an available ninth binding site for water coordination. As previously mentioned, the typical coordination number for lanthanoid complexes is 9.
- (ii) Additionally, the paramagnetic Gd3+ induces a localized magnetic field, influencing the behavior of water molecules not coordinated to the metal center.
- Chelates containing gadolinium are approximately 50 times less hazardous than the “free” Gd3+ found in salts like GdCl3. Chelates containing gadolinium usually disappear from blood plasma in a matter of hours (half-life <2 h) and are eliminated through urination. Conversely, blood serum contains free Gd3+ ions for a much longer period; just 2% are eliminated after seven days.
References
- Katjia A. Strohfeldt, Essential of Inorganic Chemistry
- Katherine H. Thompson and Chris Orvig, Lanthanide compounds for therapeutic and diagnostic applications, 2006, 35, 499-499.
- Simon P Fricker, The therapeutic application of lanthanides, 2006 Jun;35(6):524-33.
- https://www.pharmacy180.com/article/the-clinical-use-of-lanthanoids-1424/