The electrolysis of water produces hydrogen and oxygen gases. When hydrogen is required, this electrolytic process is used in some industrial applications. Electrolysis is the process of converting water (H2O) into hydrogen (H+) and hydroxide (OH–) ions by passing an electric current through it. Ions move to opposite electrodes, releasing pure hydrogen (H2) and oxygen (O2) gases. It is an oxidation-reduction reaction that does not occur spontaneously. Because heat in the form of electricity is supplied to the electrolytic cell, the reaction is endothermic.
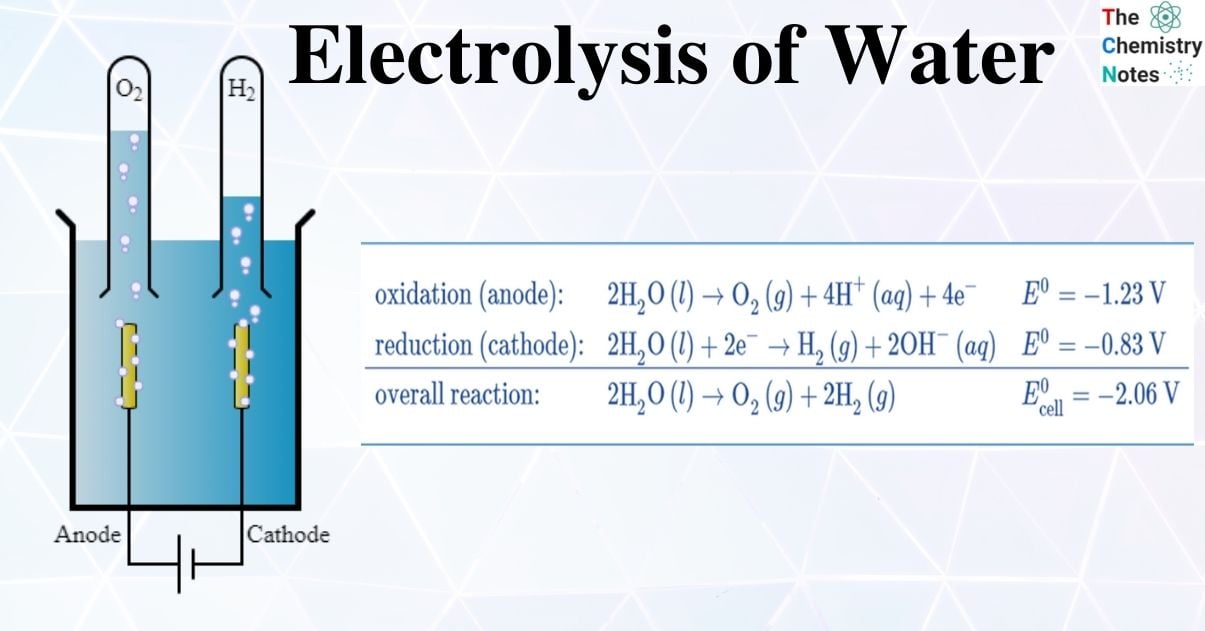
Interesting Science Videos
Electrolysis of Water
Water Electrolysis is the breakdown of water (H2O) into oxygen (O2) and hydrogen gas (H2) by passing an electric current through it. Water electrolysis, also known as electrochemical water splitting.
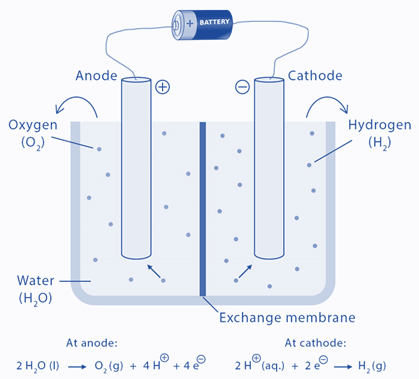
The electrolytic cell is made up of a pair of platinum electrodes immersed in water with a small amount of an electrolyte such as H2SO4. The electrolyte is required because pure water cannot carry enough charge due to a lack of ions. Water is oxidized to oxygen gas and hydrogen ions at the anode. Water is reduced to hydrogen gas and hydroxide ions at the cathode.

Water electrolysis is a popular method used in a variety of industries, most notably the food industry, metallurgy, and power plants. Furthermore, the components of water, such as hydrogen and oxygen, have numerous applications. For example, hydrogen produced by electrolysis is a clean, renewable, and efficient fuel source.
Principle of Electrolysis of Water
Two inert metal electrodes or plates, such as platinum or iridium, are submerged in water. These plates are powered by a direct current (DC) electrical source. Hydrogen will appear at the cathode (where electrons enter the water). Oxygen will appear on the anode side. In the ideal faradaic efficiency, hydrogen will be produced twice as much as oxygen. Both, however, will be proportional to the total electrical charge carried by the solution. However, in some cells, side reactions can occur, resulting in the formation of different products with less than optimal faradaic efficiency.
Spontaneity of the process
The cell potential of pure water electrolysis is negative, making it thermodynamically unfavorable. Because of the low ion concentration and the interfaces to be crossed, an extra voltage (overvoltage) of about 0.6V is required at each electrode.
However, the Gibbs free energy for the process at standard conditions is a higher positive value, approximately 474.4 kJ.
- Because of these considerations, the process is “impossible” to carry out without the addition of electrolytes to the solution and the necessary energy supplied by an external electrical power source.
- Continuous electrolysis of pure water is only possible in practice at an external voltage of 2.4 V.
- One method is to increase conductivity by increasing the number of ions available through the addition of acid, base, or non-reacting salts.
Since pure water electrolysis is thermodynamically impossible, techniques to make it possible kinetically are being researched.
Electrolyte selection
The conductivity of water increases significantly when a water-soluble electrolyte is added. The electrolyte separates into cations and anions; anions rush to the anode to neutralize the buildup of positively charged H+; similarly, cations rush to the cathode to neutralize the buildup of negatively charged OH–. This allows for an uninterrupted flow of electricity.
An electrolyte must be chosen with caution because an anion from the electrolyte competes with the hydroxide ions to give up an electron. Instead of the hydroxide, an electrolyte anion with a lower standard electrode potential than the hydroxide is oxidized, and no oxygen gas is produced. Rather than reducing a hydrogen ion, a cation with a higher standard electrode potential will do so, and no hydrogen gas will be created.
- Li+, Rb+, K+, Cs+, Ba2+, Sr2+, Ca2+, Na+, and Mg2+ have lower electrode potentials than H+ and are thus suitable for use as electrolyte cations. Sodium and lithium are frequently used because they combine to form cheap, soluble salts.
- If an acid is used as the electrolyte, the cation is H+, and there is no competitor for the H+ produced by water dissociation. Sulfate (SO4 2-) is the most commonly used anion because it is very difficult to oxidize, with the standard potential for oxidation of this ion to peroxydisulfate ion being +2.010 volts.
- Because of their high conductivity, strong acids like sulfuric acid (H2SO4) and strong bases like potassium hydroxide (KOH) and sodium hydroxide (NaOH) are frequently used as electrolytes.
- A solid polymer electrolyte, such as Nafion, can also be used, and when used in conjunction with a special catalyst on each side of the membrane, it can efficiently split the water molecule with as little as 1.5 volts.
Electrolysis of Pure Water
For the electrolysis of pure water, an excess of energy in the form of overpotential is usually required. This excess energy is critical because without it, the process moves at a glacial pace, if at all. Water’s limited self-ionization is another reason for this. Furthermore, the electrical conductivity of pure water is one millionth that of seawater. The efficiency of electrolysis can be increased by using a suitable electrolyte, such as a salt, acid, or base.
Electrolyzers
The electrolyzer is an electrolytic cell used for water electrolysis.
Electrolyzers are classified into three types based on the electrolyte transporter.
Polymer Electrolyte Membrane (PEM) Electrolyzer
A polymer, such as Nafion, separates the electrodes and allows hydrogen ions formed by water oxidation at the anode to pass through to the cathode compartment for discharge and formation of hydrogen gas.
Alkaline Electrolyzers
The use of dilute aqueous sodium (or potassium) hydroxide in electrolysis allows hydroxide ions to move to the anode and form oxygen.
Solid Oxide Electrolyzer
The electrodes are separated by ceramic oxide. Water is reduced to hydrogen and oxide ions at the cathode. The oxide ions pass through the ceramic oxide to the anode, where they are converted to oxygen gas. At high temperatures of 700 to 800 °C, this is used to reduce the external voltage required for electrolysis.
Applications of Electrolysis of Water
- The vast majority of hydrogen generated by electrolysis is a byproduct of the production of chlorine and caustic soda. This is a typical example of a competing for side reaction.
2NaCl + 2H2O → Cl2 + H2 + 2NaOH
- Water electrolysis is used to produce oxygen for the International Space Station.
- As a byproduct of the chlor alkali process, hydrogen is used to make specialty chemicals and other small-scale applications.
- This hydrogen gas can be used as hydrogen fuel or combined with oxygen to create oxyhydrogen gas, which is used in welding and other applications.
- Water electrolysis produces heavy water.
- Electrolysis generates about 5% of the hydrogen gas produced globally.
- Hydrogen is used in the Haber process to produce ammonia for fertilizer, as well as hydrocracking to convert heavy petroleum sources to lighter fractions.
References
- Zumdahl, Steven S.; Zumdahl, Susan A. (1 January 2013). Chemistry (9th ed.). Cengage Learning. p. 30. ISBN978-1-13-361109-7
- https://byjus.com/jee/water-electrolysis/
- https://en.wikipedia.org/wiki/Electrolysis_of_water#Applications
- https://www.chemistrylearner.com/electrolysis-of-water.html
- https://chem.libretexts.org/Bookshelves/Introductory_Chemistry/Introductory_Chemistry_(CK-12)/23%3A_Electrochemistry/23.09%3A_Electrolysis_ of_Water
- https://flexbooks.ck12.org/cbook/ck-12-chemistry-flexbook-2.0/section/23.10/primary/lesson/electrolysis-of-water-chem/
- https://www.chemeurope.com/en/encyclopedia/Electrolysis_of_water.html