The availability of mineral nutrients is essential for all species’ growth, but none is more crucial than nitrogen, which is needed in significant quantities since it is a necessary component of proteins, nucleic acids, and other cellular components. Nearly 79% of the nitrogen in the earth’s atmosphere is present as the gas N2, which is in abundant supply. Although essential, nitrogen gas is a limiting element. All living things, including people, plants, and other creatures, need nitrogen for daily biological functions.
Although nitrogen (N) is not a rare element on earth, it is not directly accessible to plants in its most prevalent forms (N2 gas in the atmosphere due to a triple bond between the two nitrogen atoms, making the molecule almost inert and thus N fixed in the planet’s crust and sediments). Nitrogen therefore frequently limits agricultural production, especially for crops like maize and hay that absorb a lot of N. Thus, N2 must be “fixed” (combined) as ammonium (NH4) or nitrate (NO3) ions to be utilized for growth.
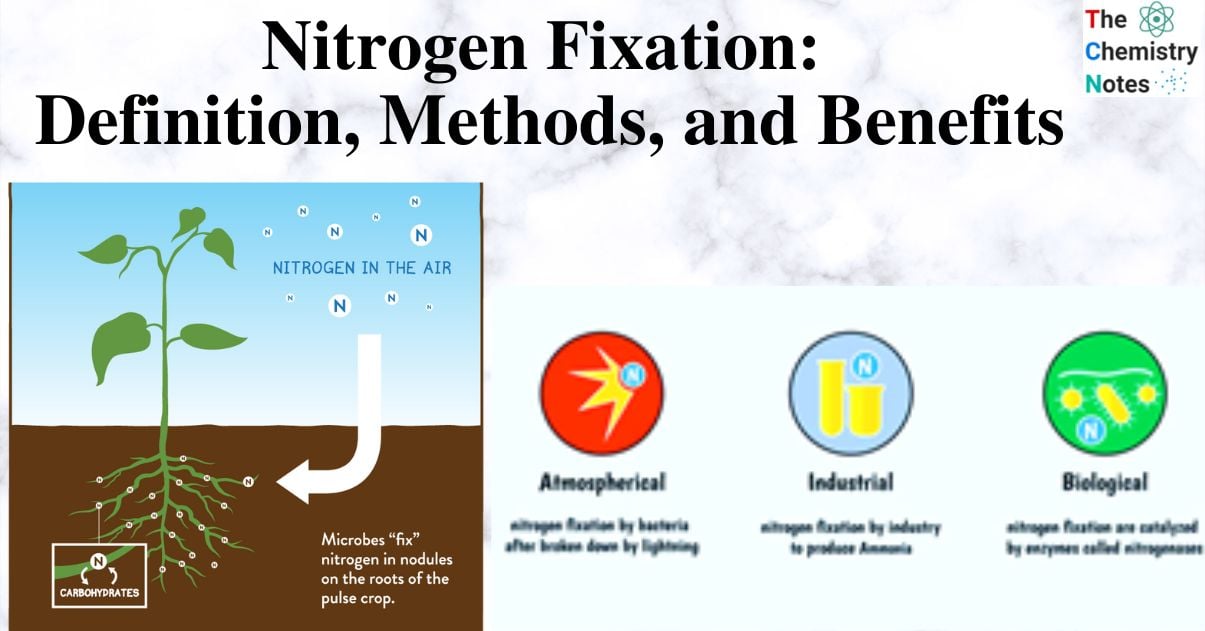
Interesting Science Videos
What is nitrogen fixation?
The conversion of atmospheric nitrogen into useful nitrogenous compounds, by natural or artificial methods, is called the fixation of nitrogen. Nitrogen present in these nitrogenous compounds is called fixed or combined nitrogen.
Methods of Nitrogen Fixation
Nitrogen is taken up by plants from the soil and used for protein synthesis. In contrast to carbon dioxide and oxygen, leaves’ stomata are unable to absorb atmospheric nitrogen due to the fact that plants cannot directly use the atmospheric nitrogen gas. In order to fix nitrogen, some bacteria and other natural phenomena along with artificial methods are required.
Natural methods for fixation of Nitrogen
A. Nitrogen fixation by lightning discharge
The transformation of nitrogen from its non-absorbable state into a useful form occurs naturally when lightning strikes. Because of the triple bond between the atoms in the N2 molecule, nitrogen in the atmosphere is extremely stable and non-reactive. Lightning generates enough heat and energy to break this bond, allowing nitrogen and oxygen atoms to combine to form NOx. the nitrogen and oxygen in the air combine to produce nitric oxide. When there is too much oxygen in the air, nitric oxide is oxidized to produce N2O5, which then combines with water to form nitric acid. Rainwater transports this acid into the soil, where it combines with the soil’s limestone and alkalies to produce nitrates, which are then stored there as plant nourishment.
N2 + O2 → 2 NO
4NO + 3O2 → 2 N2O5
N2O5 + H2O → 2HNO3
2HNO3 + CaCO3 → Ca(NO3)2 + CO2 +H2O
Even though lightning has a minor role in nitrogen fixation, it protects plants from a lack of vital nutrients.
B. Nitrogen fixation by symbiotic bacteria
A few bacterial and cyanobacterial species have the ability to fix atmospheric nitrogen, or change nitrogen gas into combined forms. By creating combined nitrogen, these bacteria can have a significant impact on soil fertility. Rhizobium is the name of the most significant genus of bacteria that fixes nitrogen. They infect root hairs and cortical cells, which leads to the formation of root nodules that serve as N fixing sites. In genral words, the symbiotic nitrogen-fixing bacteria penetrate the host plants’ root hairs, where they multiply and encourage the growth of root nodules, which are enlargements of plant cells and bacteria that are closely bonded. The bacteria in the nodules convert free nitrogen to ammonia, which the host plant uses for growth.
Rhizobium coexists symbiotically with plants in the Leguminosae family, such as peas, beans, clover, and alder trees, in the nodules on their roots. There are other, but fewer, bacteria that can fix dinitrogen in the soil close to roots. Despite the fact that plants need nitrates, bacteria in the soil are able to easily turn other nitrogenous substances into nitrates.
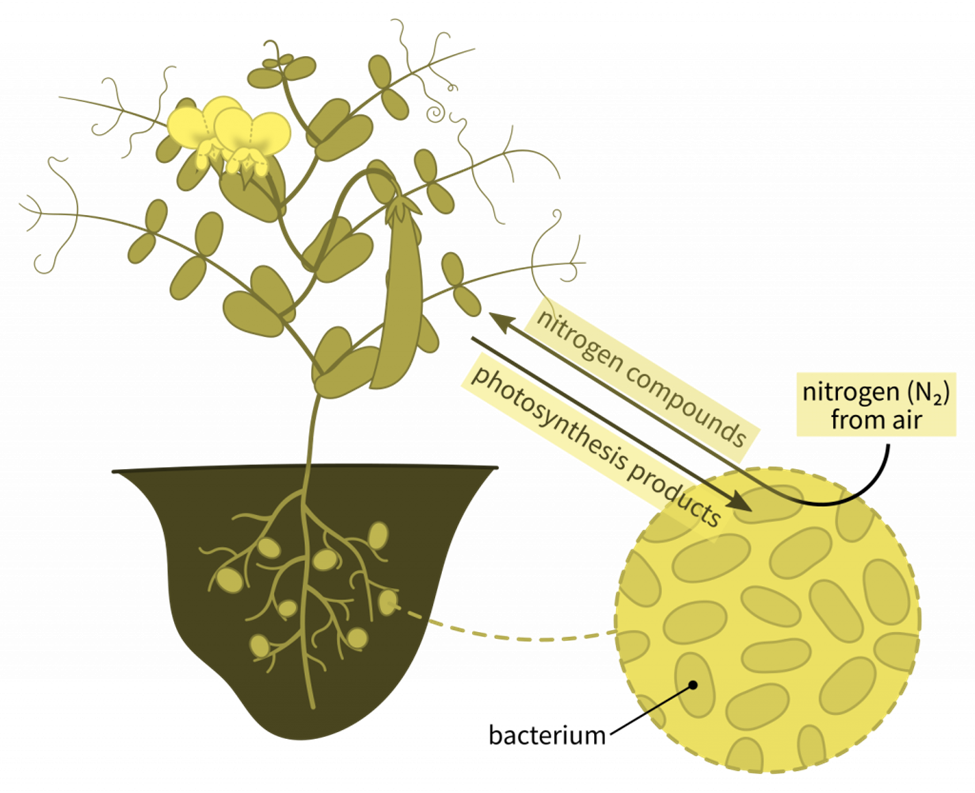
The non-absorbable nitrogen form is changed into a useful form throughout this biological process. Plants take up the modified nitrogen from the soil once it has dissolved in the soil in this form. This is the reason for farmers’ implementing crop rotation, in which legume plants replenish soil nitrogen levels without the use of fertilizers.
Artificial methods used for the fixation of Nitrogen
Nitrogen fixing occurs gradually through natural processes. In order to meet our ever-increasing need, chemists have created techniques for generating nitrogen molecules used as fertilizers. Today, artificial fixation processes provide more than half of the nitrogen molecules that the world needs.
Some of the common artificial methods employed for the fixation of Nitrogen are:
A. Haber’s process
This is the landmark process in artificial nitrogen fixation as Haber’s technique results in a significant amount of atmospheric nitrogen being transformed into ammonia. In the presence of a catalyst (Fe + Mo), nitrogen and hydrogen combine at the proper temperature (450°C) and pressure (200-900 atmospheric pressure) to give ammonia.

B. Birkland and Eyde Process
It is one of the first processes employed to manufacture nitrogen-based fertilizers. During the process, nitric oxide is created by forcing airborne nitrogen to react with oxygen under the impact of an electric arc at a temperature of 3000–3500°C. Nitrogen dioxide is created when this oxide mixes with more oxygen. Nitric acid is created when the dioxide is dissolved in water while being exposed to oxygen or air.
N2 + O2 → 2NO
2NO + O2 → 2NO2
4NO2 + O2 + 2H2O → 4HNO3
C. Cyanamide Process
When nitrogen gas, produced by evaporating liquid air, is passed over calcium carbide that has been heated to 1100oC, the two combine to produce a mixture of calcium cyanamide and carbon, often known as nitrolim, which is widely used as fertilizer.
CaC2 + N2 → CaCN2 + C (Nitrolim)
CaCN2 + 3H2O → CaCO3 + 2NH3
D. Ostwald’s Process
It is another useful method for the artificial fixation of nitrogen. This method turns ammonia into nitric acid. Oxygen and ammonia are combined, and the mixture is then run over a platinum catalyst that has been heated.
E. Nitride process
When certain metals, including magnesium and aluminum, are heated in a nitrogen-rich environment, the corresponding nitrides are produced. Then, the nitride combines with the water to produce ammonia.
3Mg + N2 → Mg2N2
2Al + N2 → 2AlN
AlN + 3H2O → Al (OH)3 + NH3
F. By using transition metal complex catalyzed system
Finding a transition metal catalyzed system that can absorb nitrogen and create ammonia for fertilizers affordably and without the need for high temperatures or pressure is of great research interest. In 1965, ruthenium trichloride was reduced with hydrazine to produce the pentaamine(dinitrogen)ruthenium cation, the first dinitrogen complex. Other techniques have also been discovered, such as replacing a complex’s labile ligand with N2. Almost all of the transition elements now have dinitrogen complexes.
Nitrogenase and its role in nitrogen fixation
The essential enzyme for biological nitrogen-fixing is nitrogenase. Rhizobium, which has nitrogenase-containing metalloenzymes, is the most well-known dinitrogen-fixing bacterium. The process of turning nitrogen into ammonia is catalyzed by nitrogenase. The nitrogenase group of enzymes is responsible for catalyzing all biological processes involving the nitrogen fixation process. Numerous kinds of nitrogen-fixing bacteria contain one or more of the following three forms of nitrogenase: Iron-only (Fe) nitrogenase, Molybdenum (Mo) nitrogenase, and Vanadium (V) nitrogenase. Molybdenum (Mo) nitrogenase is the most studied and characterized among these three forms.
General Characteristics of Nitrogenase Enzyme
- It is mainly found in prokaryotes and helps in nitrogen fixation in the living organisms.
- It’s a protein called Mo-Fe. There are two alpha and two beta subunits in this heterotetramer.
- In the nitrogenase enzyme, Mo is in the oxidized state (III).
- Molecular oxygen makes it very sensitive. The anaerobic environment is necessary for the functioning of the nitrogenase enzyme.
- Between the beta and alpha subunits, it also has Fe-S clusters.
- The respiration of host cells provides the ATP needed for the transformation of nitrogen into ammonia.
- As an oxygen scavenger, the leg-haemoglobin found in the nodules aids in maintaining the anaerobic conditions necessary for the nitrogenase enzyme’s activity.
This enzyme catalyze the conversion of dinitrogen into ammonia. The full reaction catalyzed by this enzyme is given below:
N2 + 8e– + 8H+ + 16MgATP → 2NH3 + H2 + 16MgADP + 16Pi
The process needs ATP to give the essential energy. The iron protein and the molybdenum-iron protein, which together make up nitrogenase, are connected by an electron transport chain. The molybdenum-iron protein receives electrons from this chain and transfers them there. The Mo-Fe protein can then lower dinitrogen levels in addition to a number of substrates.
Two identical subunits make up the iron protein. It is generally agreed that the Fe protein contains four Fe and four S2-, which can be extruded as a single [4Fe-4S] cluster, and that it has two binding sites for MgATP. It is assumed that the [4Fe-4S] functions to carry electrons to the Mo-Fe protein.
Benefits of using Biological Nitrogen fixation
- Biological nitrogen fixation reduces the cost of production with better yields.
- By using inoculants as N fertilizer substitutes, water resource contamination issues caused by leaching and runoff of excess fertilizer are avoided.
- In many regions, microorganisms boost the productivity of legume crops. Even when production increases are not apparent, Biological nitrogen fixation phenomenon frequently boosts the protein content of the edible seeds of legumes.
- By Nitrogen fixing legumes, non-legume crops can benefit from increased soil fertility, permeability, and organic matter through techniques including green manuring, crop rotations, and alley cropping.
- Legume inoculants can be produced or distributed with a low level of energy. Compared to applying fertilizer to the field, applying seed is easier. Through Biological nitrogen fixation, long-term leguminous tree crops are self-sustaining.
Denitrification
Denitrification is the process by which the nitrogen component is released back into the atmosphere by turning nitrate (NO3–) into gaseous nitrogen (N). It is regarded as the reverse of nitrification or nitrogen fixation and is responsible for the reduction of the amount of fixed nitrogen present in the soil. Denitrification occurs naturally in controlled ecosystems such as marine and freshwater habitats, tropical and temperate soils, wastewater treatment facilities, aquifers, manure stockpiles, etc., and is a universal process for both terrestrial and aquatic ecosystems.
Thiobacillus species and Pseudomonas bacteria found in the soil carry out the denitrification process when there is no oxygen available. This process results in the generation of nitrous oxide (N2O) and nitrogen gas, which are eventually released into the atmosphere, from nitrate compounds found in soil and aquatic systems. Since large number of micro-organisms are involved, it is called microbial mediated process.
Numerous bacteria use oxygen instead of nitrate for respiration when the oxygen (O2) supply in the soil is depleted. When there is a lack of oxygen for respiration or when the soil is damp, moist, or submerged in water, denitrification is most likely to occur. Even while some fungi can denitrify, they are not thought to be important.
- In this process, soil microorganisms transform nitrogen (N) gases that are lost from the soil into plant-available soil nitrate (NO3–).
- Nitric oxide (NO), nitrous oxide (N2O), and dinitrogen (N2) are among the gases produced during denitrification.
Nitrite → Nitric Oxide → Nitrous oxide → Nitrogen gas.
In areas where more than 60% of the soil’s pore space is filled with water, denitrification is more prevalent.
Factors affecting Denitrification
Denitrification is also influenced by some the factors like temperature, soil pH, soil texture, moisture content in the soil, oxygen content in the soil, the concentration of nitrate in the soil, and so on. However, the amount of organic matter in the soil has a major impact on the denitrification process. The only source of food for the bacteria is the organic materials present in the soil. Soil bacteria need a supply of easily accessible organic material, which can come from the soil, plants, or other sources.
The microbial community and soil conditions both affect the final gas. Microbes carry out their tasks by converting more nitrate to dinitrogen (N2) gas as oxygen deficiency worsens. Denitrification causes a loss of valuable nitrogen (N) for nutrient management purposes, although the effect on the atmosphere will differ.
References
- https://chem.libretexts.org/Bookshelves/Environmental_Chemistry/Supplemental_Modules_(Environmental_Chemistry)/Biochemical_Cycles/Nitrogen_Cycle#:~:text=Nitrogen%20in%20the%20air%20becomes,it%20into%20ammonia%2C%20NH3.
- https://www.britannica.com/science/nitrogen-cycle
- Postgate J (1998). Nitrogen Fixation (3rd ed.). Cambridge: Cambridge University Press.