Known more often as radiopharmaceuticals, radiopharmacy is the production and administration of radioactive materials for use as radioactive medications. Tools for therapy or diagnosis can be employed with radiopharmaceuticals. The use of radiopharmaceuticals as therapeutic and diagnostic tools has drawn researchers to create new radioligands for tracking, staging, and assessing the course of disease as well as the effects of radiation treatment and/or conventional therapy.
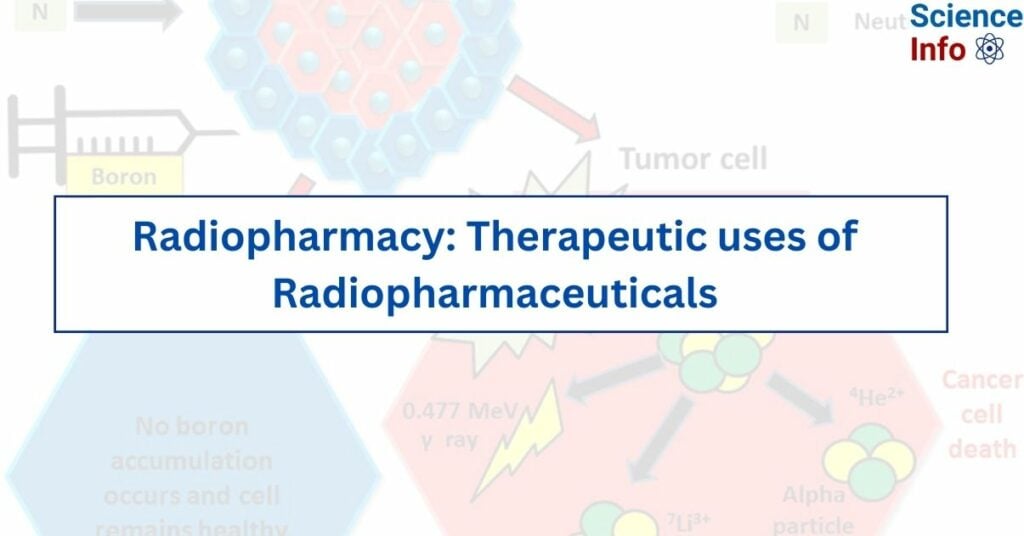
Interesting Science Videos
What does radiopharmaceutical mean?
- Radiopharmaceuticals, which consist of both radionuclidic and pharmaceutical components, are medicinal substances containing radioactive elements (radioisotopes).
- In recent times, there has been a notable rise in the utilization of radiopharmaceuticals for both diagnostic and therapeutic purposes. Various strategies have been employed to formulate therapeutic radiopharmaceuticals, playing pivotal roles in the field of nuclear medicine.
- Presently, the application of therapeutic radiopharmaceuticals extends to the treatment of diverse conditions such as thyroid cancer, metastatic bone cancer, neuroendocrine tumors, and myeloproliferative disorders through radioimmunotherapy.
- These treatments offer multiple conveniences and present potential advantages when compared to alternative therapeutic approaches.
- The evolving landscape of radiopharmaceuticals underscores their increasing significance in medical interventions.
Radiopharmacy: dispensing and protection
Radiation therapy should minimize any negative effects on the patient’s health when using radioactive materials for diagnostics. As a result, low half-lives and exclusively emitting γ-radiation are thought to be optimum for radioactive elements.
- Since the radioactive 99mTc exclusively produces γ-rays and has a 6-hour half-life, it is frequently employed in conjunction with a gamma camera for imaging applications. For therapeutic purposes, radionuclides that release β-particles are more suited. Thyroid illnesses that spread to other parts of the body and hyperthyroidism are treated with 131I and its β-radiation. Gamma radiation, which is also released by 131I, is useful for diagnosing renal function and precisely determining the glomerular filtration rate.
- Humans can suffer from sickness, skin burns, cancer, sterility, hair loss, and even death as a result of radiation exposure. However, each of these adverse consequences is contingent upon the radiation type, energy, penetrating power, and duration of exposure.
- Radiation has several beneficial uses when applied properly. These include the dating of archeological artifacts, the production of energy, the treatment of cancer, and the sterilization of medical equipment.
The safe handling of radioactive material depends on having the appropriate radiation shielding. Radiation protection can be accomplished via shielding; lead or tungsten is required to properly shield from gamma rays, while plastic and aluminum can shield from β rays. In addition, the duration and distance of exposure play a significant role in providing adequate radiation protection. The square of the distance from the radiation source determines the radiation dose in an inverse manner. Reducing the duration of exposure also lowers the possibility of radiation side effects.
Diagnosis of Diseases with Radiopharmaceuticals
In nuclear medicine, gamma-ray-emitting radiopharmaceuticals are used to diagnose diseases and assess the health and function of particular tissues and organs. Radiopharmaceuticals are used in two main procedures for illness diagnosis:
- Non-image diagnostic applications: The targeted organ or tissue is exposed to the radiopharmaceutical, and the radioactivity is measured externally. The Medical Internal Radiation Dose (MIRD) can be used to quantify the radioactivity in the organ, and Monte Carlo techniques can be used to compute the body surface area.
- Image diagnostic applications: When the radiopharmaceutical reaches the targeted organ or tissue, it is scanned using a variety of imaging systems, including gamma cameras, PET/CT, SPECT/CT, single-photon emission computed tomography (SPECT), and PET/CT hybrid systems.
How does radiopharmaceutical work?
- Radiopharmaceuticals belong to a category of medications employed in the treatment of metastatic pheochromocytoma, a vascular tumor of the adrenal gland, as well as paraganglioma, rare tumors found near the carotid artery and along nerve pathways in the head and neck.
- They are also utilized to address neuroendocrine tumors, alleviate pain in individuals with osteoblastic metastatic bone lesions, and serve as diagnostic agents in thyroid function evaluation and the detection of thyroid malignancies.
- Additionally, radiopharmaceuticals play a crucial role in lymphatic mapping, aiding in the identification of lymph nodes draining from primary tumor sites in patients with solid tumors.
- These pharmaceuticals consist of radioactive isotopes bound to biological molecules, enabling them to target specific organs, tissues, or cells within the human body for both diagnostic and therapeutic purposes.
- Through the emission of radiation, they effectively target tumors by employing molecules carrying radioactive particles that bind to specific receptors expressed by the tumor. This dual functionality underscores the versatility and significance of radiopharmaceuticals in the realm of medical diagnostics and treatments.
“Scintigraphy” is the term for the process of creating images of organs or tissues using radiopharmaceuticals. A particular kind of medical equipment called a gamma camera can recognize the gamma rays that the radioisotope emits and produce non-invasive images that show how the organ or tissue being studied functions. Because they are used to diagnose malfunction in bodily tissues, radiopharmaceuticals are often referred to as radiotracers. Oral and injectable administration are used to give radiopharmaceuticals.
Therapeutic uses of radiopharmaceuticals
Molecules having radiolabelling are known as radiopharmaceuticals and are utilized therapeutically. This indicates that some of the atoms in this molecule have had their radioactive isotopes swapped out for them. These radiolabelled compounds are intended to target specific illness areas across the body with therapeutic doses of ionizing radiation, primarily β-radiation. There should be fewer adverse effects when the targeting is done. It is crucial to take into account the radionuclide’s decay characteristics as well as the clearance pathway and rate from nontarget radiosensitive tissue when designing a therapy regimen that includes radiopharmaceuticals.
131Iodine for therapy of hyperthyroidism
- I is the chemical symbol for iodine, and its atomic number is 53. It belongs to group 17 of the periodic table of elements, which contains the halogens.
- The vapor of elemental iodine has a purple color that defines it. Like the other halogens, free iodine is usually found as the diatomic molecule I2. The anion that is most soluble in water is called iodide (I−), and it is mostly found in oceans. Nutrition is the primary application for iodine and its constituents.
- Due to its ease of incorporation into organic compounds and relatively low toxicity, it is frequently used as a component of X-ray contrast agents.
- Because humans need iodine to manufacture thyroid hormones, iodine will build up in the thyroid gland. Iodine contains multiple radioactive isotopes in addition to its single stable isotope (127 53I).
- A portion of these are utilized in medicine, such as in diagnostic procedures and therapeutic interventions. Iodine radioisotopes can be employed therapeutically because they will accumulate in the thyroid gland. 129I, 125I, and 123I are radioactive isotopes with half-lives of 15.7 million years, 59 days, and 13 hours, respectively.
- The last one’s short half-life and gamma radiation make it useful as an imaging agent in nuclear medicine.
- Areas of radioisotope buildup in the human body can be captured on camera using a gamma camera.
- In cases of neuroblastoma and pheochromocytoma, I-131-MIBG is quite successful. Furthermore, paraganglioma, carcinoid tumors, and medullary thyroid carcinoma respond less well to I-131-MIBG. Pregnant individuals should not get this treatment. For the treatment to be successful, bone marrow reserve and renal functioning should be adequate.
Nuclear fission, as observed during the Chernobyl accident, produced 131I, a radioisotope that emits β and is carried to the thyroid gland through inhalation. The radioisotope can be substituted by treating it with nonradioactive potassium iodide. However, when administered in large dosages, 131I can be utilized as a therapeutic agent against thyroid cancer. Paradoxically, tiny dosages of the β-emitting radioisotope promote cancer, while high enough levels will destroy the surrounding tissue. Consequently, 131I–containing medicines are frequently utilized to treat hyperthyroidism. Usually, these formulations are taken orally as a solution or as capsules.
Therapeutic use of 89Strontium
- With an atomic number of 38 and a group 2 designation on the periodic table, strontium is an alkaline-earth element with the chemical symbol Sr.
- Compared to calcium, strontium is a soft, gray metal that reacts more with water. It releases hydrogen gas and strontium hydroxide when it comes into contact with water.
- Most commonly, strontium metal is stored under mineral oil to shield it from oxidation. Four stable isotopes—84Sr, 86Sr, 87Sr, and 88Sr—combine to make natural strontium, with the latter being the most common.
- The synthetic radioisotope 89Sr has a half-life of 50.5 days and emits beta radiation. It is produced when 88Sr is activated by neutrons and decays to the stable state of 89yttrium.
- An FDA-licensed product that contains 89Sr is called Metastron. It has a 28-day expiration date and comes in a vial that is ready to use. It comes with a calibration vial so the pharmacist can make sure the patients receive the precise dosage that is prescribed.
- Due to the close resemblance between strontium and calcium, which are neighboring elements in the periodic table, it is hypothesized that strontium undergoes metabolic processes in the human body like calcium, leading to its accumulation, particularly in bones. This characteristic has prompted the utilization of strontium as a therapeutic approach for alleviating pain associated with bone metastasis, a prevalent issue affecting over 50% of patients with prostate, breast, or lung cancer.
- The specific mechanism through which bone pain is relieved remains unclear. The intravenous administration of 89SrCl2 results in rapid clearance from the bloodstream, mirroring the distribution pattern of calcium, and subsequent deposition in the bone mineral. Strontium tends to localize within the hydroxyapatite cells of the bones rather than in the bone marrow cells. The radioisotope 89Sr emits localized β-radiation, generating a pain-relieving effect. A significant portion of the administered SrCl2 actively targets the metastatic sites. Any unbound SrCl2 is eliminated through renal excretion or excreted along with feces.
- Patients receiving 89SrCl2 are most likely to experience low platelet counts as a side effect. After six months of treatment, platelet counts ought to revert to their pre-administration levels. Patients who already have low platelet or white blood cell counts should not receive therapy with 89SrCl2, and even after the treatment is finished, blood parameters for these patients need to be monitored regularly.
177Lutetium dotatate treatment
- lutetium-177 Dotatate, a radiopharmaceutical that is given for the treatment of gastroenteropancreatic neuroendocrine tumors (GEP-NETs) is dotatate.
- This radiopharmaceutical uses beta-radiation therapy to treat cancer while focusing on the structure of the tumor. This type of treatment is administered four or five times over two months on average.
- Vital organs such as the liver, kidney, and bone marrow are exposed to a certain amount of dose throughout this treatment. The patient’s weight and the maximal treatment dose are taken into account while adjusting the radiation dose in the target organ.
- Neuroendocrine tumors primarily affect the colon, stomach, pancreas, and lungs. Lu-177 dotatate and I-131-metaiodobenzylguanidine (MIBG) are now utilized to treat neuroendocrine carcinoma.
Boron neutron capture therapy (BNCT)
- There are 14 radioisotopes with extremely short half-lives and two stable isotopes of boron, 10B and 11B. The most common isotope, 11B, accounts for 80% of natural boron, while 10B (about 20%) is used extensively in the so-called boron neutron capture therapy (BNCT).
- BNCT (Boron Neutron Capture Therapy) stands out as a noninvasive treatment alternative for malignant tumors, particularly those affecting the brain and head and neck regions, currently undergoing clinical trials. In this approach, the patient receives an injection of a nonradioactive compound containing 10B, serving as a neutron-capturing agent with a notable affinity for cancerous tissues. Once the compound reaches the tumor site, the patient is subjected to a stream of low-energy neutrons, known as epithermal neutrons.
- Despite losing energy upon penetrating the skin, these neutrons retain the capacity to interact with the neutron-capturing agent, triggering a nuclear reaction. The outcome of this reaction involves the conversion of 10B to the nonradioactive isotope 7Li, accompanied by the emission of low-energy gamma radiation and α-radiation (4 2He2+ particles). α-Radiation possesses a limited range and directly targets the local tumor tissue from within the tumor cells. The linear energy transfer (LET) of these α-particles spans approximately one cell diameter, ensuring minimal exposure to healthy tissue. BNCT represents a promising avenue for cancer treatment with its focus on precision and selectivity in targeting malignant cells. (10B + nth → [11B]→4He+7Li + 2.31MeV)
- Numerous carrier molecules for 10B, including amino acids, carbohydrates, antibodies, and liposomes, have been studied. As of right now, clinical investigations are only using two boron compounds as BNCT delivery vehicles. While the boron-based amino acid (l)-4-dihydroxy-borylphenylalanine (BPA, boronophenylalanine) is utilized in clinical trials in Europe and the United States, sodium mercaptoundecahydro-closo-dodecaborate (Na2B12H11SH), also known as borocaptate (BSH), was primarily employed in clinical trials in Japan.
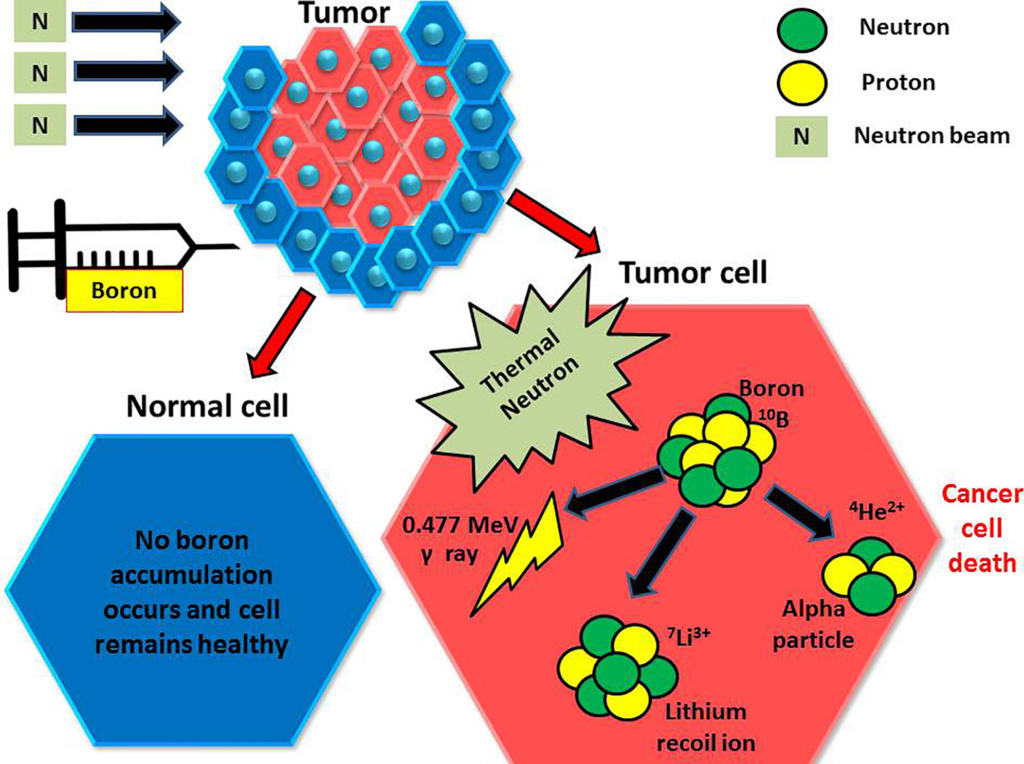
Radiopharmaceuticals in Theranostics Administration
Theranostics primarily find application in treating cancerous and infected regions, offering a blend of diagnostic and therapeutic attributes. By harnessing both functions, theranostics effectively mitigate the side effects of treatments, fostering enhanced patient compliance and increased survival rates. This innovative approach involves the integration of molecular imaging, where diagnostic and therapeutic techniques are coupled with a diagnostic agent possessing a chemical structure identical or similar to the therapeutic agent.
Through this methodology, valuable insights into treatment response predictions are gleaned. Diseases can be categorized based on their molecular phenotype, enabling the observation of the molecule’s biodistribution and facilitating the monitoring of treatment response. This integrated approach exemplifies a dynamic paradigm in healthcare, allowing for refined disease classification and personalized treatment strategies.
References
- Katjia A. Strohfeldt, Essential of Inorganic Chemistry
- Craig W. Lindsley, Christa E. Müller, and Salvatore Bongarzone, Diagnostic and Therapeutic Radiopharmaceuticals, J. Med. Chem. 2022, 65, 19, 12497–12499.
- Erol AKGUN , Emre OZGENC , Evren GUNDOGDU, Therapeutic Applications of Radiopharmaceuticals: An Overview, J. Pharm. Sci., 46, 1, 93-104, 2021.
- https://www.rxlist.com/radiopharmaceuticals/drug-class.htm