According to the second law of thermodynamics, the entropy of an isolated system or any cyclic process never decreases; it either increases or remains constant. As a result, the second law establishes a definite direction in which time must progress by stating that time can only pass in the direction of increasing entropy. This addresses a problem with determining the direction of time solely through observation. Specifically, most processes, particularly those occurring on a microscopic scale, are symmetric with time: they appear the same in reverse as they do when time passes normally. Observing the process alone will not tell you which way time is moving.
It asserts that there cannot be a process that reduces the entropy of an isolated system and that time always moves the universe from one space-time plane of lower entropy to one of equal or greater entropy. The latter is the basis for the thermodynamic study of the second law.
Interesting Science Videos
What is Second Law of Thermodynamics?
The second law of thermodynamics is a statement that describes the amount of useful work that can be done by a heat-exchange or heat-transfer process.
This law can be stated precisely in two ways, as originally proposed in the nineteenth century by the Scottish physicist William Thomson (Lord Kelvin) and the German physicist Rudolf Clausius.
According to the second law of thermodynamics, any spontaneously occurring process will always result in an increase in the universe’s entropy (S). In simple terms, the law states that the entropy of an isolated system will never decrease over time.
Nonetheless, when a system is in thermodynamic equilibrium or is undergoing a reversible process, the total entropy of the system and its surroundings remains constant.
The second law is also known as the Law of Increased Entropy.
Second Law of Thermodynamics Key Points
- When a hot object comes into contact with a cold object, heat flows from the hotter to the colder, never from colder to hotter on its own. Heat could still be conserved if it left the cooler object and went to the hotter one. That is where the second law of thermodynamics comes into play.
- The entropy of an isolated system can never decrease over time and is constant if and only if all processes in nature are reversible. The systems are isolated and evolve spontaneously towards thermodynamic equilibrium, the state with the greatest entropy.
- In ideal cases where the system is in thermodynamic equilibrium or in an undergoing reversible process in nature, the entropy of the total surroundings and the system can remain constant. All processes, including spontaneous processes, increase the total entropy of the system and its surroundings, and the process is irreversible in nature and in the thermodynamic sense. The increase in entropy accounts for the irreversibility of natural processes and the asymmetry between past and future.
Heat Engines, Heat Pumps, and Refrigerators
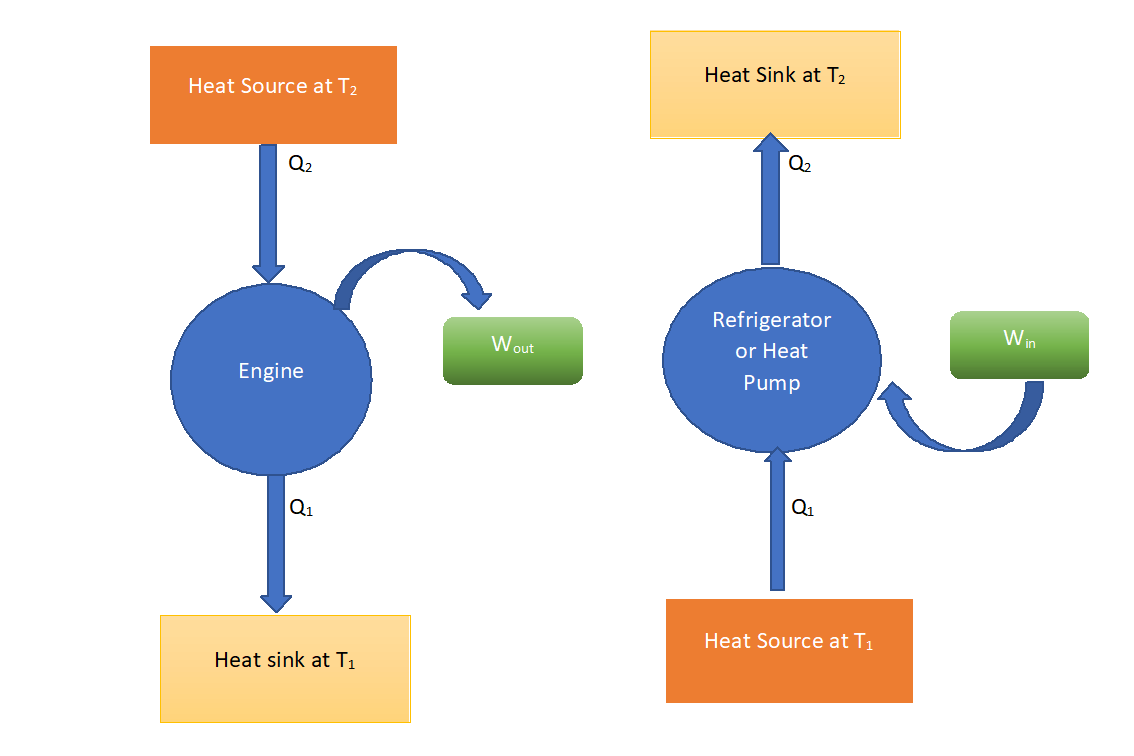
To generate work, heat is transferred from a heat reservoir to an ideal heat engine.
Unfortunately, transferring heat to a heat engine without the use of a working fluid is nearly impossible. As a result, if the working fluid is fed into the heat engine it must be released into the environment with no heat or at zero absolute temperature. This is nearly impossible to accomplish, so any realistic heat engine will need to operate between two heat reservoirs, one to provide heat and the other to store it and another to absorb the working fluid after it has done its job.
W = QH + QL
Here, H and L respectively denotes for high and low temperatures.
Heat engines
It is impossible to extract a certain amount of heat (Q2) from a hot reservoir and use it entirely to perform work (W). A portion of the heat (Q1) must be exhausted to a cold reservoir. This rules out a perfect heat engine.
This is known as the Kelvin-Planck statement of the second law and is sometimes referred to as the “first form” of the second law.
Refrigerators and Heat Pumps
Heat cannot flow from a colder body to a warmer body without any work being done to accomplish this flow. Energy does not naturally flow from a low temperature object to a higher temperature object. This rules out a perfect refrigerator. The same principles apply to air conditioners and heat pumps as they do to refrigerators.
This is the “second form,” also known as the Clausius statement of the second law.
There are basically three devices that operate in a cycle that can be represented by this model of a heat engine. These are a true heat engine, a heat pump, and a refrigerator.
For the true heat engine, the objective is to produce work, so its efficiency is given by
ⴄ = W/QH
Note that Q=W + QL and h could be optimized by minimizing QL. The heat pump absorbs work and produces heat to the upper reservoir. Its coefficient of performance (COP) is given by
(COP)HP = QH/W
Once again, QH = W + QL, but COP could be maximized by minimizing W. The refrigerator absorbs work and removes heat from the low-temperature reservoir. Its coefficient of performance is given by
COPR = QL/W
In this case, COP could be maximized by also minimizing W.
Statements of Second Law of Thermodynamics
There are famous two statements of Second Law of Thermodynamics. They are:
Kelvin-Planck Statement
“It is impossible to construct a device which operates on a cycle and
produces no other effect than the production of work and the transfer of heat from a single body.”
The statement by Kelvin-Planck is based on the fact that the efficiency of the heat engine cycle is never 100%. This means that some heat is always rejected to the low temperature reservoir during the heat engine cycle. The heat engine cycle always produces work by operating between two heat reservoirs.
An engine, for example, can absorb heat and convert it into work, but only if it completes a cycle. Without completing a cycle, the substance in the engine is not in its original state, resulting in a “other effect.” Another example is a gas chamber that can absorb heat from a heat reservoir while also performing isothermal work against a piston as it expands. However, if the gas were to be returned to its original state (i.e., made to complete a cycle), it would have to be compressed and heat extracted from it.
The Kelvin statement exemplifies a well-known engineering problem. Despite advances in technology, we are unable to create a heat engine that is 100% efficient.
Clausius Statement
One of the earliest statements of the Second Law of Thermodynamics was made by R. Clausius in 1850. He stated the following-
“It is impossible to construct a device which operates on a cycle and whose sole effect is the transfer of heat from a cooler body to a hotter body”.
It is impossible to build a device that can transfer heat from a colder body to a warmer one without consuming any work. Furthermore, energy does not spontaneously flow from a low-temperature object to a higher-temperature object. It is critical to understand that we are discussing the net transfer of energy. Energy can be transferred from a cold object to a hot object through the transfer of energetic particles or electromagnetic radiation. In any spontaneous process, however, the net transfer will occur from the hot object to the cold object. And work is required to transfer the net energy to the hot object. In other words, unless the compressor is powered by an external source, the refrigerator will not function. Clausius statement is used by the heat pump and refrigerator.
The Clausius and the Kelvin-Planck statements have been shown to be equivalent
Applications of Second Law of Thermodynamics
- Heat always flows from a body at a higher temperature to a body at a lower temperature, according to the law. This law applies to all types of heat engine cycles, including Otto, Diesel, and others, and to all working fluids used in the engines. This law has resulted in the advancement of modern vehicles.
- Refrigerators and heat pumps based on the Reversed Carnot Cycle are another application of this law. If you want to move heat from a lower temperature body to a higher temperature body, you must supply external work. Heat generates work in the original Carnot Cycle, whereas heat is transferred from a lower temperature reservoir to a higher temperature reservoir in the Reversed Carnot Cycle.
- Thermodynamic laws govern both air conditioners and heat pumps. The air conditioner removes heat from the room and keeps it at a lower temperature by dissipating it into the atmosphere. The heat pump absorbs heat from the environment and transfers it to the room, which is cooler in the winter.
- Thermodynamics is also used in biological systems. The best example is the production of ATP. The energy stored in ATP bonds powers the synthesis of glucose-6-phosphate. Compounds with a high phosphate group transfer potential include acyl phosphate, enol phosphate, and others.
Limitations of Second Law of Thermodynamics
The Second Law of Thermodynamics has the following limitations:
- This law only addresses the irreversibility of heat conversion.
- The second law of thermodynamics applies only to closed systems.
References
- https://chem.libretexts.org/Bookshelves/Physical_and_Theoretical_Chemistry_Textbook_Maps/Supplemental_Modules_(Physical_and_Theoretical_Chemistry)/Thermodynamics/The_Four_Laws_of_ Thermodynamics/ Second_Law_of_Thermodynamics
- https: //byjus.com/jee/second-law-of- thermodynamics/
- https:// www.toppr.com/ guides/ physics/thermodynamics/second-law-of-thermodynamics/
- R. Chang, “Physical Chemistry for the Chemical and Biological Sciences”, University Science Books, Sausalito, California (2000).
- Aston, J. and Fritz, J.J., Thermodynamics and Statistical Mechanics, John Wiley and Sons, Inc., New York, 1959.
- Atkins, P.W. and Julio de Paulo, Atkins’ Physical Chemistry, Oxford University Press, UK, Indian Edition 9, 2011.