The activation energy (Ea) refers to the quantity of energy necessary for a successful collision between particles, leading to the breaking of bonds and the production of products. The presence of activation energy is a prerequisite for the initiation of a reaction, which elucidates the reason behind the non-spontaneous occurrence of certain exothermic reactions at ambient temperature.
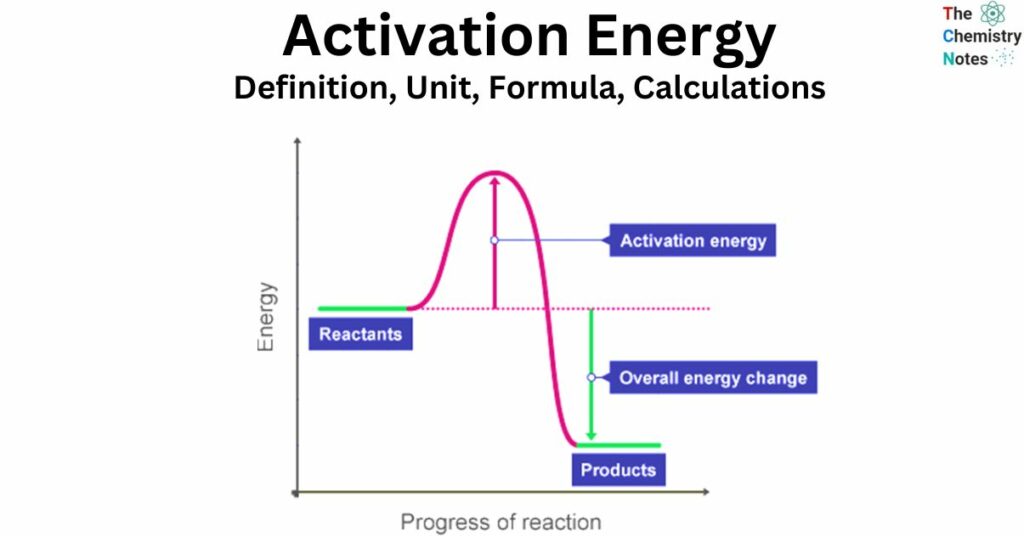
Interesting Science Videos
What is Activation Energy?
Activation energy refers to the minimum energy required by the particles involved in a specific reaction in order for the reaction to occur.
In order for particles to undergo a reaction, it is necessary for them to collide with enough energy to provide the activation energy. Without this energy, the particles will not react. Activation energy is a prerequisite for any reaction to occur.
In order to initiate a chemical reaction, it is necessary to break the bonds present in the reactants. This process demands the input of energy. Activation energy refers to the amount of energy required to initiate a reaction.
Unit of Activation Energy
Activation energy, commonly represented as Ea, is usually measured in joules (J) and or kilojoules per mole (kJ/mol) or kilocalories per mole (kcal/mol).
Factors Affecting Activation Energy
Activation energy depends on two factors.
Nature of Reactants
For ionic reactants, the value of (Ea) is low because of the attraction between the reactant and the reacting species. In contrast, for a covalent reactant, the Ea value is high due to the significant energy required to break the existing bonds.
Catalyst Effect
The positive catalyst provides an alternate path in which the value of Ea is low, whereas the negative catalyst creates an alternate path in which the value of Ea is high, and vice versa.
Why is activation energy important in Chemistry?
Activation energy is important because it determines the rate at which a chemical reaction occurs. It is the hurdle that reactions must overcome to occur. Knowing the required energy is crucial for ensuring a successful reaction.
Let’s use boiling water as an example. Setting your stove to 90 °C will prevent your water from boiling. Using a Bunsen burner to heat a flask with reactants would also prevent the reaction from occurring. Activation energy is crucial for reactions to occur.
The formula for Activation Energy
We employ the Arrhenius equation to determine activation energy:
The Arrhenius equation demonstrates how the activation energy, temperature, and frequency factor of a reaction affect its speed.
Activation Energy and Rate of Reaction
The rate of a reaction relates to the activation energy.
When the activation energy is high, the reaction moves more slowly because fewer reactants have enough energy at any given time to break through the energy barrier. If the activation energy is high enough, a process won’t happen at all unless energy is added. For example, burning wood gives off a lot of energy, but a table made of wood doesn’t quickly catch fire. For wood to burn, it needs energy to start, which can come from a lighter.
The Arrhenius equation shows how the rate of a process, the activation energy, and the temperature are related.
k = Ae-Ea/(RT)
Here, k is the reaction rate coefficient, A is the frequency factor for the reaction, e is the irrational number (about 2.718), Ea is the activation energy, R is the universal gas constant, and T is the exact temperature (in Kelvin).
The Arrhenius equation shows that the rate of a chemical process changes as the temperature does. Most of the time, chemical processes go faster (up to a point) as the temperature goes up. In some cases, the rate of a response slows down as the temperature goes up.
Activation Energy Calculation
The Arrhenius equation describes the relationship between reaction rate, activation energy, and temperature.
We know the formula for rate constant is given by,
k = Ae−EaRT
Taking log on both sides we get,
ln k = −EaRT + ln A
For initial temperature T1 and rate of constant k1, the equation is written as,
ln k1 = −Ea/RT1 + ln A …….. (1)
For the final temperature T2 and rate of constant k2, the equation is written as,
ln k2 = −Ea/RT2 + ln A …….. (2)
Subtracting (2) from (1) we get,
ln k2 – ln k1 = −Ea/RT2 + ln A − (− Ea/RT1 + ln A)
ln (k2/k1) = EaR (1/T1 − 1/T2)
2.303 log (k2/k1) = EaR (1/T1 − 1/T2)
Ea = 2.303 R (log k2/k1) [T1T2 / (T2 – T1)]
This derives the formula for activation energy.
Graphical Representation of Activation Energy
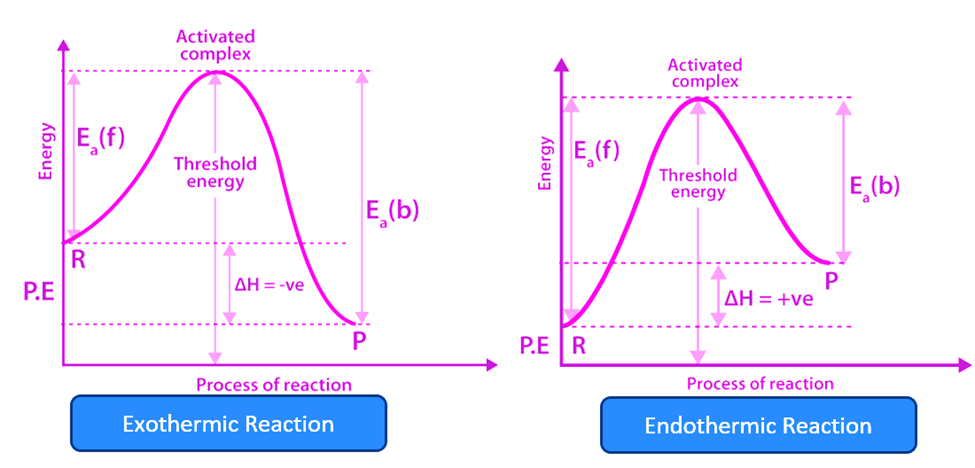
From the reactants to the top of the graph is where the activation energy is calculated. An endothermic process (right) requires substantially larger activation energy than an exothermic reaction (left).
For an endothermic reaction, the products have more energy than the reactants, and it is generally preferable for a system to have as little energy as feasible. This is why endothermic reactions require the addition of energy/heat to the system.
Activation Energy: How Does It Work?
To understand how activation energy works, you need to know what happens during a chemical process. The reactants change into products. A new bond is formed when an old one is broken. Before the bonds break, the molecules change shape and reach an unstable high-energy state. To reach this state, the molecules must be given energy. This energy is called the activation energy, and the state that changes quickly is called the transition state. In the transition state, the molecules form an active complex. Due to their instability, however, molecules do not spend much time there. They go to a lower-energy state and move on to the next step of the reaction or make stable products.
The energy of the transition state is greater than that of the reactants or the products. So, whether the response goes forward or backward, the activation energy is always positive. For a forward process, the activation energy is the difference in energy between the transition state and the reactants. It is the difference in energy between the transition state and the products for a reaction that goes in the opposite direction.
The enthalpy change (ΔH), also called the heat of the reaction, is equal to the difference between the energy of the products and the energy of the reactants. For a chemical process to happen, energy must also be added. It is also true for exergonic reactions, in which energy is eventually given off, leading to a negative change in enthalpy.
Is Negative Activation Energy Possible?
An elementary reaction has an activation energy of zero or positive. A reaction mechanism with numerous steps, though, might have a negative activation energy. In situations when the rate of reaction slows as the temperature rises, the Arrhenius equation also allows for negative activation energy values.
Barrierless reactions are elementary reactions with negative activation energies. In these situations, raising the temperature reduces the likelihood that reactants may combine as a result of having too much energy. It’s comparable to hurling two sticky balls at one another. When moving slowly, they stick, but when moving quickly, they collide and bounce.
Changes in Activation Energy Caused by Catalysts
A catalyst is a material that speeds up a chemical reaction without being depleted in the process. In order to reduce the activation energy of processes, such compounds alter the transition states involved. Enzymes are sort of catalysts that are made up entirely of proteins and sometimes other tiny molecules.
Catalysts can decrease the activation energy of a reaction as a whole, but they have no effect on the initial energies of the reactants or the final energies of the products.
Gibbs Energy and Activation Energy
Another relation describing reaction rate is the Eyring equation. Transition state Gibbs energy, rather than activation energy, is used in the equation. Both the enthalpy and the entropy of a reaction are included in the Gibbs energy of the transition state. Although they are connected, activation energy and Gibbs energy cannot be substituted for one another in chemical equations.
Free energy | Activation energy |
It is represented as ∆G because free energy is also called Gibbs free energy. | Free energy is the amount of energy that can be used to carry out the thermodynamic process. |
It is represented as ∆G, because free energy is also called Gibbs free energy. | It is represented as Ea. |
No effect of catalysts. | Effect of catalysts. |
Activation energy can be represented as k=Ae-EaRT; where k is the rate constant, A is the Arrhenius constant, R is the gas constant, and Ea is the activation energy. | Activation energy can be represented as k=Ae-EaRT; where k is the rate constant, A is the Arrhenius constant, R is the gas constant, Ea is the activation energy. |
Video on Activation Energy
Frequently Asked Questions (FAQ)
Can a Catalyst change the activation energy?
By lowering the activation energy, the catalysts can speed up the chemical process. It is usually a chemical that is added to speed up the process without getting used up in the reaction.
Positive and negative catalysts are the most common types. A positive catalyst speeds up a process by lowering the activation energy, while a negative catalyst slows it down by raising the activation energy.
How are free energy and activation energy different?
Free energy is the energy that can be used to do work in a thermal system. On the other hand, activation energy is the energy barrier that a chemical process has to get past in order to continue and make products
What would happen if there were no activation energy barriers?
If there were no activation energy barrier, chemical processes would happen on their own.
Does temperature affect activation energy?
No, the temperature does not affect activation energy. A catalyst is needed to lower the activating energy.
What is the significance of calculating activation energy?
All chemical processes, including exothermic ones, require activation energy to start. Reactants require activation energy to move together, overcome repulsion forces, and initiate bond-breaking.
References
- https://www.chemistrylearner.com/activation-energy.html
- https://chemed.chem.purdue.edu/genchem/topicreview/bp/ch22/activate.php
- https://www.bbc.co.uk/bitesize/guides/z2gccdm/revision/4
- https://unacademy.com/content/jee/study-material/chemistry/factors-affecting-activation-energy/
- https://collegedunia.com/exams/formula-of-activation-energy-chemistry-articleid-1414
- https://chemistrytalk.org/catalysts-activation-energy/