The composition of a reaction product can be determined by thermodynamic and kinetic control in the presence of competing reactions that yield different products under varying reaction conditions.
Typically, two factors have an impact on the potential outcome of a reaction.
- The thermodynamic factors, specifically the relative stability of the products, are being considered.
- The kinetic factors, specifically the rate of product formation, are under consideration.
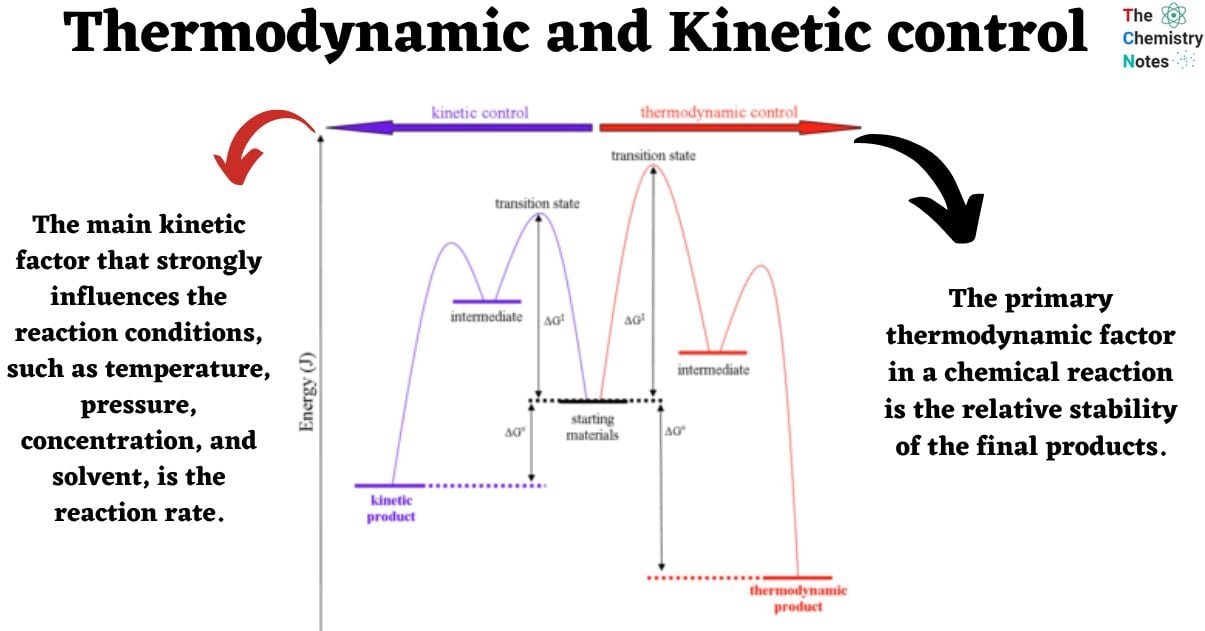
Interesting Science Videos
What is Thermodynamic and Kinetic control?
“Kinetic product” refers to the product that is formed at a faster rate, while the conditions that lead to the formation of the kinetic product are referred to as “kinetic control.”
“Thermodynamic product” is used to describe the product that is formed at a faster rate, while the term “Thermodynamic control” refers to the conditions that lead to the formation of the kinetic product.
The principle of kinetic control dictates that the product with the lowest activation energy, Ea, and the fastest formation rate is favored in a chemical reaction.
The principle of thermodynamic control dictates that the product with the highest level of stability is favored.
Difference between Thermodynamics and Kinetic
The field of kinetics pertains to the measurement and analysis of the rate at which a chemical reaction occurs. Thermodynamics pertains to the stability of reactants and products. The inquiry that arises is whether there exists a correlation between kinetics and thermodynamics. The interrelation between kinetics and thermodynamics is evident in various aspects. Let’s take the following reaction, for example, as an example:
aA + bB → c AB
The stoichiometric constants of reactant A and reactant B are denoted by a and b, respectively, while the stoichiometric constant of the product AB is represented by c. The stoichiometric coefficient, also known as a constant, refers to the numerical value placed before a chemical species in a balanced equation.
The thermodynamic quantity known as the chemical equilibrium constant, Keq, is determined by taking the ratio of the products, raised to the power of the stoichiometric coefficients of the balanced equation, to the reactants, raised to the power of the stoichiometric coefficients. The equilibrium constant for the aforementioned reaction can be determined as follows:
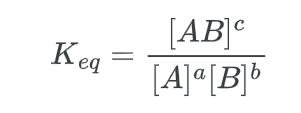
Alternatively, the initial kinetic rate law for this reaction can be represented by the instantaneous rate law.

where the negative sign denotes a decrease in the concentrations of the reactants ([A] and [B]).
In general, chemical reactions tend to remain far from reaching chemical equilibrium for a prolonged duration. Three factors will determine the outcome of chemical reactions in the laboratory.
- The rate at which products are formed.
- The stability of the products in relation to each other.
- The conditions for the reaction, including temperature, pressure, concentration, and solvent.
- In order to control the product mixture for a given experiment, one can adjust the reaction conditions and manipulate the Gibbs free energy difference, ΔG.
Chemists have the ability to control the reaction mechanism, whether it be thermodynamic or kinetic, in order to enhance the production of the intended product. It is essential to engineer the mechanism of control for a chemical reaction, distinguishing between thermodynamic and kinetic control. The control mechanism has a dual impact. It not only influences the desired product yield for a given reaction mixture but also determines the reaction pathway that is followed.
Selectivity determines the pathway that a chemical reaction takes. The selectivity of a reaction is determined by the control mechanism, which involves manipulating the difference in Gibbs free energy, ΔG. The selectivity of a reaction is determined by the molecular mechanism of the reaction.
The difference in Gibbs free energy, ΔG, can be expressed as:
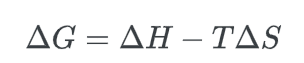
The equation involves three variables: ΔH, which represents the enthalpy difference, T, which represents the temperature, and ΔS, which represents the entropy difference.
- Enthalpy, denoted by H, refers to the amount of potential energy that is stored within a chemical bond. The enthalpy difference, represented by ΔH, refers to the variation in potential bond energy that exists between the reactants and products involved in a chemical reaction.
- Entropy, denoted by the symbol S, is a measure of the degree of disorder or randomness in a chemical system.
The characterization of a reversible reaction as either spontaneous or non-spontaneous is relevant in the following ways:
A spontaneous reaction occurs when the products have a lower free energy level than the reactants. A thermodynamically favorable reaction, also known as a spontaneous reaction, occurs without requiring external energy input from the surroundings.
A non-spontaneous reaction occurs when the free energy level of the reactants is lower than that of the products. A reaction that is non-spontaneous or thermodynamically unfavorable can only take place with the addition of external energy from the surroundings.
The energy of activation, Ea, represents the kinetic barrier for both spontaneous and non-spontaneous reactions.
- The activation energy, denoted as Ea, refers to the minimum amount of energy that reactants must possess in order to transform into products.
- In most reactions, energy input from the surroundings is necessary to create an unstable and high-energy intermediate chemical species known as the transition state.
- If a reaction pathway has a lower activation energy, it will produce a kinetic product that is thermodynamically favorable.
Finally, it should be noted that at equilibrium, the products exhibit the highest level of thermodynamic stability and the difference in Gibbs free energy is zero, represented by ΔG = 0.
Energy diagram of Thermodynamic and kinetic control
The following simple reaction coordinate diagram provides a basis for the key issues about thermodynamic and kinetic control:
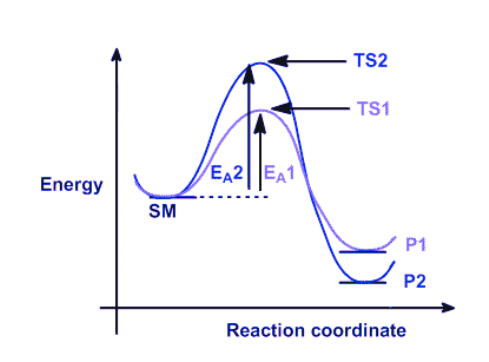
The example under consideration involves a starting material, denoted as SM, which can undergo a reaction leading to the formation of two distinct products, namely P1 and P2. The formation of these products occurs through competing pathways.
- The production of product 1 (P1) occurs through transition state 1 (TS1) via pathway 1 in reaction 1. Given that this reaction has a lower energy (more stable) transition state and a lower activation barrier, it will occur more quickly. Hence, it can be inferred that P1 represents the kinetic product, i.e., the product that is formed at the highest rate.
- Product 2 (P2) is produced through transition state 2 (TS2) via pathway 2 in reaction 2. The stability of P2 can be attributed to its lower energy state in comparison to P1. Thus, it can be inferred that P2 represents the thermodynamically favored product, which is characterized by greater stability.
Thermodynamic vs Kinetic Control in Organic Reactions
In organic chemistry, the idea of kinetic vs. thermodynamic regulation of reactions is crucial. Many known processes have two (or more) potential reaction products, but when the reaction is conducted at a low temperature, one of the results—the kinetic product—predominates. When the reaction is carried out at a higher temperature, the other (thermodynamic product) dominates.
Adding hydrogen bromide (HBr) to 1,3-butadiene
Upon addition of HBr to 1,3-butadiene, a mixture of 3-bromo-1-butene (resulting from 1,2-addition) and 1-bromo-2-butene (resulting from 1,4-addition) is formed.
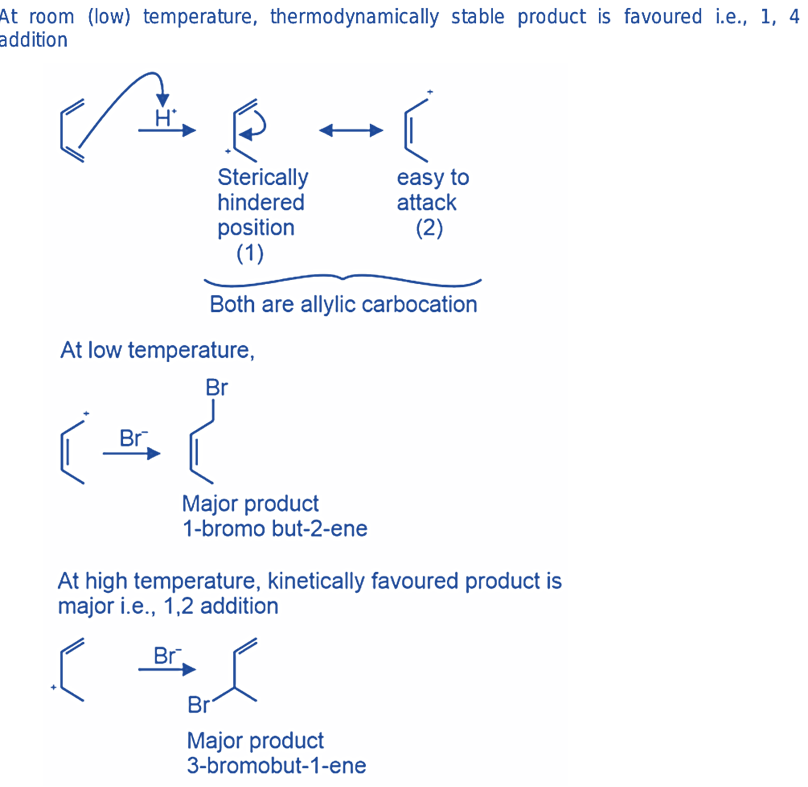
The predominant product at a temperature of -80 oC is 3-bromo-1-butene, whereas, at a temperature of 40 oC, 1-bromo-2-butene is the principal product.
Under kinetic conditions, it is observed that nucleophilic attack by bromide takes place at the secondary allylic carbon instead of the primary allylic carbon. The heats of formation calculations confirm that the thermodynamic product, namely 1-bromo-2-butene, exhibits greater stability than 3-bromo-1-butene.
Thermodynamic and Kinetics Requirements of a Reaction
The requirements of a reaction in terms of thermodynamics and kinetics are essential factors to consider.
- The primary thermodynamic factor in a chemical reaction is the relative stability of the final products.
- The main kinetic factor that strongly influences the reaction conditions, such as temperature, pressure, concentration, and solvent, is the reaction rate.
Video on Thermodynamic vs Kinetic Control
References
- https://chem.libretexts.org/Bookshelves/Organic_Chemistry/Organic_Chemistry_(Morsch_et_al.)/14%3A_Conjugated_Compounds_and_Ultraviolet_Spectroscopy/14.03%3A_Kinetic_vs._Thermodynamic_Control_of_Reactions
- https://library.fiveable.me/ap-chem/unit-9/thermo-kinetic-control/study-guide/hRZ0V3goVueXCw1JeUdA
- Organic Chemistry, 3rd ed., M. A. Foxe & J. K. Whitesell, Jones & Bartlett, 2004 ISBN 0-7637-2197-2
- A Guidebook to Mechanism in Organic Chemistry, 6th Edition, Peter Sykes, Pearson Prentice Hall, 1986. ISBN 0-582-44695-3
- https://lms.su.edu.pk/lesson/895/kinetic-control-vs-thermodynamic-control
- https://www.studysmarter.co.uk/explanations/physics/thermodynamics/thermodynamic-and-kinetic-control/