Thermodynamics is the branch of physics that studies the relationship between heat and other properties of a substance (such as pressure, density, temperature, and so on). Thermodynamics, in particular, focuses on how heat transfer is related to various energy changes within a physical system undergoing a thermodynamic process. In general, thermodynamics is concerned with the transfer of energy from one location to another and from one form to another. The key idea is that heat is a type of energy that corresponds to a specific amount of mechanical work. The laws of thermodynamics govern such processes, which usually result in the system doing work.
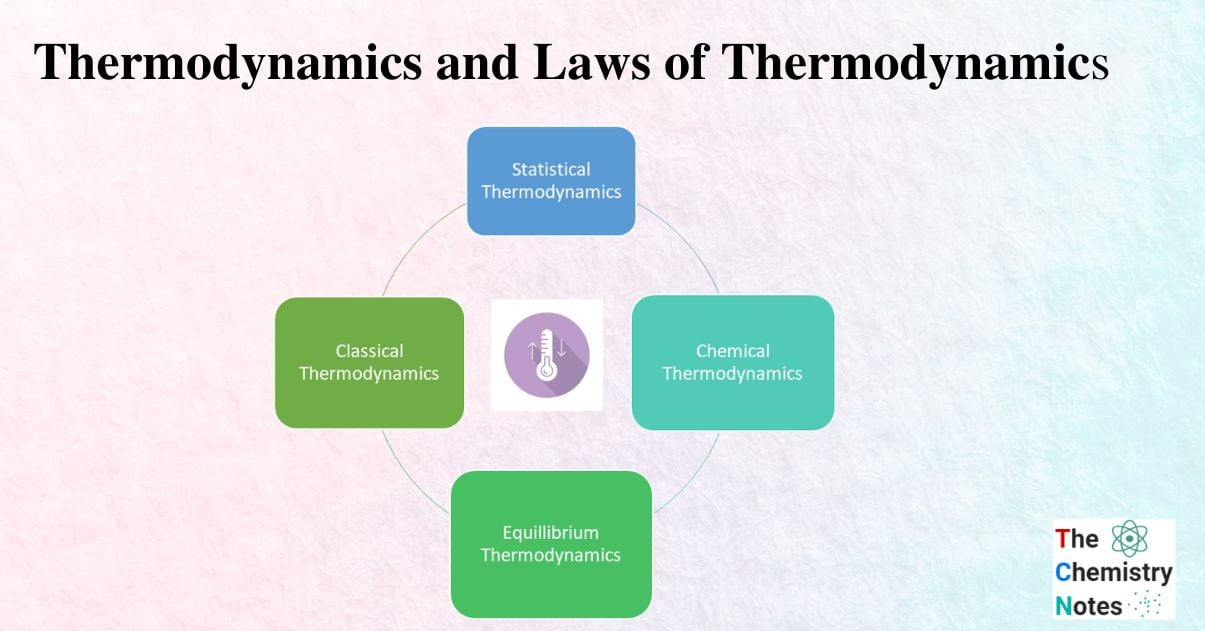
Interesting Science Videos
Basic Concepts of Heat Transfer
Heat transfer refers to any or all of several types of phenomena, referred to as mechanisms, that transport energy and entropy from one location to another. In general, heat is understood to be a representation of the energy contained within the particles of a material. This is known as the kinetic theory of gases, though the concept also applies to solids and liquids to varying degrees. The heat generated by the motion of these particles can transfer into nearby particles, and thus into other parts of the material or other materials, via several mechanisms:
- Thermal contact occurs when two substances can impact each other’s temperature.
- Thermal equilibrium occurs when two substances in thermal contact no longer transfer heat.
- Thermal expansion occurs when a substance expands in volume as it absorbs heat. There is also thermal contraction.
- Conduction occurs when heat flows through a heated solid. The transfer of energy through a solid material is known as conduction. It occurs when two bodies come into direct contact and molecules transfer energy across the interface.
- Convection occurs when heated particles transfer heat to another substance, such as cooking something in boiling water. Convection is the transfer of heat to or from a fluid medium. Molecules in a gas or liquid in contact with a solid body transmit or absorb heat to or from that body and then move away, allowing other molecules of the fluid to move into place and repeat the process.
- Radiation occurs when heat is transferred via electromagnetic waves, such as those emitted by the sun. Radiation is the emission of energy through electromagnetic (EM) radiation, specifically infrared photons carrying heat energy.
- Insulation is the use of a low-conductivity material to prevent heat transfer.
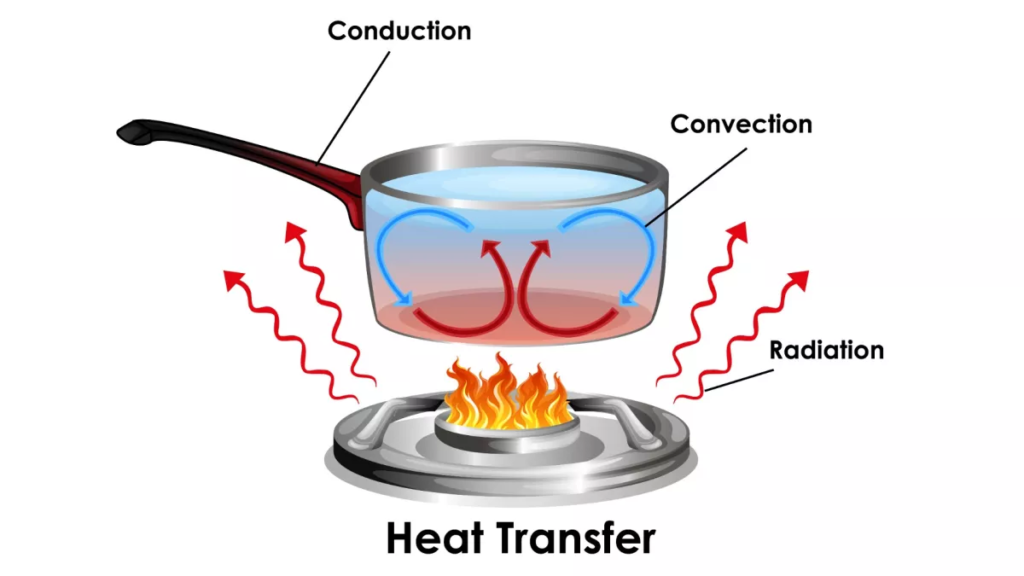
Branches of Thermodynamics
Thermodynamics is classified into following four branches:
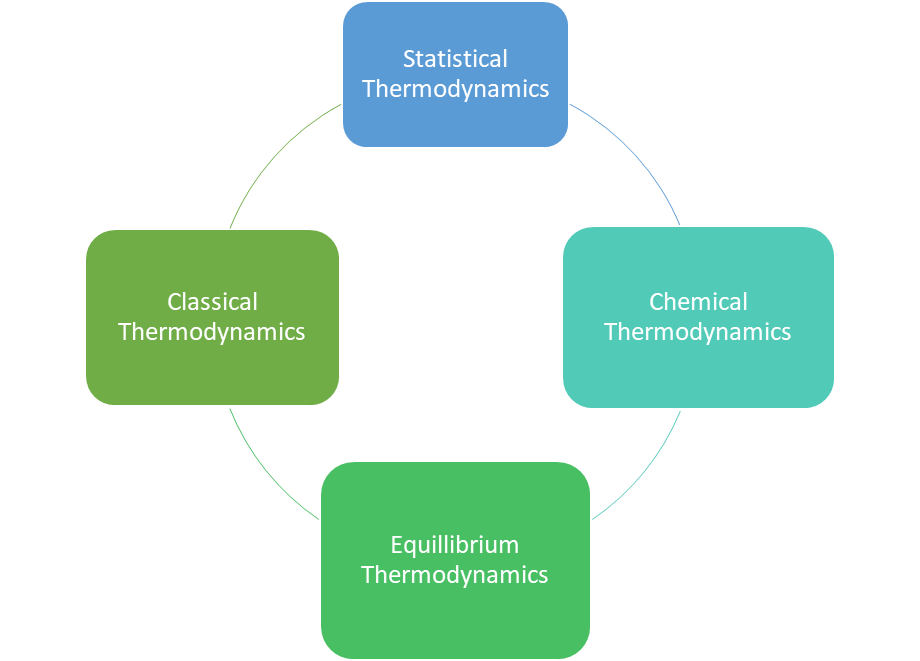
- Classical Thermodynamics: The behavior of matter is studied using a macroscopic approach in classical thermodynamics. Temperature and pressure are taken into account, allowing individuals to calculate other properties and predict the characteristics of the matter undergoing the process.
- Statistical Thermodynamics: Every molecule is investigated in statistical thermodynamics, which means that the properties of each molecule and how they interact are used to characterize the behavior of a group of molecules.
- Chemical Thermodynamics: Chemical thermodynamics is the study of how work and heat relate to each other in chemical reactions and in changes of states.
- Equilibrium Thermodynamics: Equilibrium thermodynamics is the study of energy and matter transformations as they approach equilibrium.
Fundamental Concept of Thermodynamics– Thermodynamic Terms
Before dwelling deeper into the insights of thermodynamics, you all need to be familiar with the basic terms related to thermodynamics.
Thermodynamic Systems
A system that is delimited from the surroundings by real or hypothetical boundaries is known as a thermodynamic system. A thermodynamic system refers to that part of the universe in which observations are made, and the remaining universe constitutes the surroundings. The surroundings contain everything other than the system. The system and the surroundings together make up the universe.
A thermodynamic system is encased in its surroundings, with which it can exchange heat and perform work. It transfers heat to its surroundings via a boundary. The boundary is the wall that separates the system from its surroundings. Thermodynamic systems can exchange energy or matter with their surroundings while also undergoing internal transformations.
The universe = The system + The surroundings
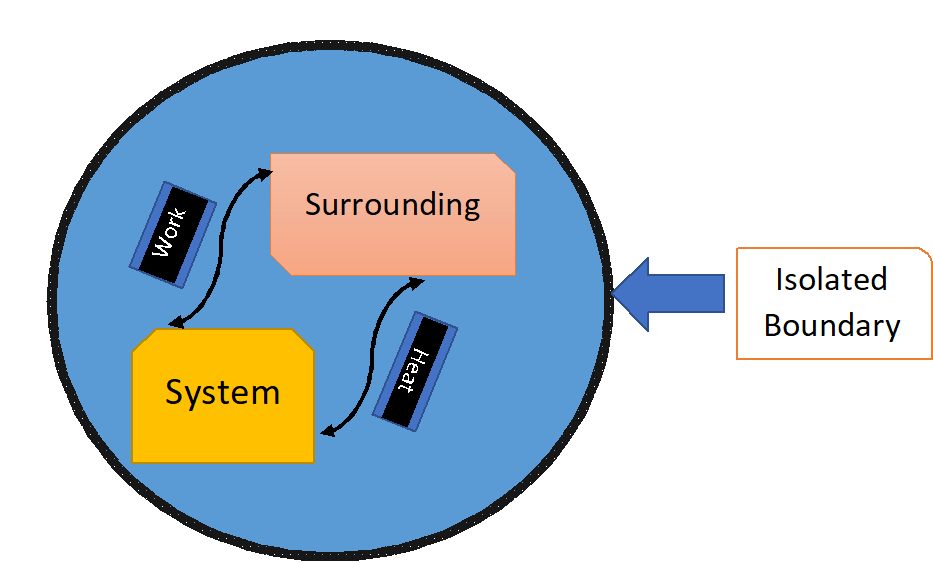
Surrounding : Everything outside the system that has a direct influence on the system’s behavior is referred to as a surrounding.
The area of matter with a distinct boundary that receives our attention is called a thermodynamic system. The system boundary can be of four types:
- Fixed boundary
- Movable boundary
- Real boundary
- Imaginary boundary
Types of System
Systems are classified into three types:
Isolated System: An isolated system is one that cannot exchange energy or mass with its surroundings. The universe is thought to be an isolated system.
Reactants are present in a thermos flask or an insulated vessel with no exchange of energy or matter with the environment. An isolated system is created when the piston and cylinder arrangement in which the fluid, such as air or gas, is compressed or expanded is insulated.
Closed System: The transfer of energy across the boundary of a closed system occurs but the transfer of mass does not. Closed systems include refrigerators and gas compression in piston-cylinder assemblies. Because the material of the vessel is conducting in nature, reactants placed in a closed vessel made of materials such as steel, copper, and silver are an ideal example of a closed system.
A closed system is represented by a cylinder with the valve closed. The mass of the cylinder does not change when it is heated or cooled.
Open System: Mass and energy can both be transferred between the system and its surroundings in an open system. An open system is exemplified by a steam turbine. Consider a beaker that contains reactants in an open beaker. The boundary in this case is an imaginary surface that encloses the beaker and reactants.
Thermodynamic Processes
A thermodynamic process occurs when there is an energetic change within a system, which is typically associated with changes in pressure, volume, internal energy (i.e. temperature), or any type of heat transfer.
There are several types of thermodynamic processes with unique properties:
- Adiabatic process – a process with no heat transfer into or out of the system.
- Isochoric process – a process with no change in volume, in which case the system does no work.
- Isobaric process – a process with no change in pressure.
- Isothermal process – a process with no change in temperature.
Thermodynamic Characteristics
Thermodynamic properties are defined as system characteristics that can specify the system’s state. These properties can be broad or narrow. Basically, they are grouped in two categories:
- Intensive Properties
- Extensive Properties
Intensive properties are those that are independent of matter quantity. Temperature and pressure are both intensive properties.
In the case of extensive properties, their values are determined by the system’s mass. Volume, energy, and enthalpy are all very broad properties.
Thermodynamic Equilibrium
All properties of a system have fixed values at any given state. As a result, if the value of even one property changes, the system’s state changes. When an equilibrium system is isolated from its surroundings, no changes in the value of its properties occur.
- Thermal equilibrium: When the temperature remains constant throughout the system.
- Mechanical equilibrium: When there is no change in pressure at any point in the system.
- Chemical equilibrium: When the chemical composition of a system does not change over time.
- Phase equilibrium: When the mass of each phase reaches an equilibrium level in two-phase systems.
If a thermodynamic system is in chemical equilibrium, mechanical equilibrium, and thermal equilibrium, and the relevant parameters no longer vary with time, it is said to be in thermodynamic equilibrium.
Thermal Expansion
Because higher temperatures cause more molecular motion, heat causes materials to expand. Because each material has its own coefficient of expansion, any change in length (or area, or volume) is proportional to the change in temperature.
Internal Energy
It is the amount of energy that a system stores within itself. This energy represents the system’s total energy. It could include any type of energy, such as kinetic or potential energy. We are aware of energy transformations. We understand that we can only transfer energy, not create or destroy it.
Basic thermodynamic terms give us an idea of the energy change associated with a chemical reaction system. When heat enters or exits a system, the internal energy of that system may change. Work can be said to be done on the system or by the system. We can also say: matter enters or exits the system.
Work
The total amount of energy that a system and its surroundings exchange within themselves is defined as work done by thermodynamics. The amount of work done is determined by external factors in the environment. These factors could include an external force, changes in temperature, pressure, or volume, and so on.
Heat
Heat is defined in thermodynamics as energy in motion. It is the energy that the kinetic energy of the substance’s molecules possessed. Heat and thermodynamics are important in assisting process designers and engineers in optimizing their processes.
It also enables them to economically harness the energy associated with chemical reactions. Heat flows from higher to lower temperatures. This concept aids scientists in the development of various heat engines.
Heat Capacity
The heat capacity, C, of an object is the ratio of heat change (energy change, Q, where the Greek symbol Delta, denotes a change in quantity) to temperature change (T).
C = Δ Q / Δ T
The heat capacity of a substance indicates how quickly it can be heated up. A good thermal conductor has a low heat capacity, which means that a small amount of energy causes a large temperature change. A good thermal insulator would have a high heat capacity, indicating that a large amount of energy is required to change the temperature.
Entropy
Entropy is a thermodynamic quantity whose value is determined by a system’s physical state or condition. In other words, it is a thermodynamic function that is used to calculate the degree of randomness or disorder.
The entropy of a solid, for example, is less than the entropy of a gas, where the particles will fill the container if they are free to move.
Enthalpy
Enthalpy is a thermodynamic system’s measurement of energy. It is the total heat content of a system, which is equal to the system’s internal energy plus the product of volume as well as pressure.
The enthalpy, H, of a system equals the sum of its internal energy, E, and the product of its pressure, P, and volume, V.
H = E + PV
Laws of Thermodynamics
Here’s only the overview of Thermodynamic laws.
Zeroth Law of Thermodynamics – Two systems each in thermal equilibrium with a third system are in thermal equilibrium to each other.
First Law of Thermodynamics – The change in the energy of a system is the amount of energy added to the system minus the energy spent doing work.
Second Law of Thermodynamics – It is impossible for a process to have as its sole result the transfer of heat from a cooler body to a hotter one.
Third Law of Thermodynamics – It is impossible to reduce any system to absolute zero in a finite series of operations. This means that a perfectly efficient heat engine cannot be created. rium with a third system are in thermal equilibrium to each other.
References
- https://byjus.com/physics/thermodynamics/
- https://www.toppr.com/guides/chemistry/thermodynamics/application-of-thermodynamic-terms/
- https://chem.libretexts.org/Bookshelves/General_Chemistry/Book%3A_ChemPRIME_(Moore_et_al.)/15%3A_Thermodynamics-_Atoms_Molecules_and_Energy/15.04%3A_Internal_Energy
- https://www.livescience.com/50776-thermodynamics.html#section-heat-transfer
- https://www.thoughtco.com/thermodynamics-overview-2699427