The stress at which a material starts to undergo plastic deformation instead of elastic deformation is known as yield strength. It is among the most crucial mechanical characteristics of a material. It assists engineers in selecting suitable materials for various applications by helping them to understand how a material deforms when stressed. Tensile testing is used to ascertain the yield strength of a material specimen.
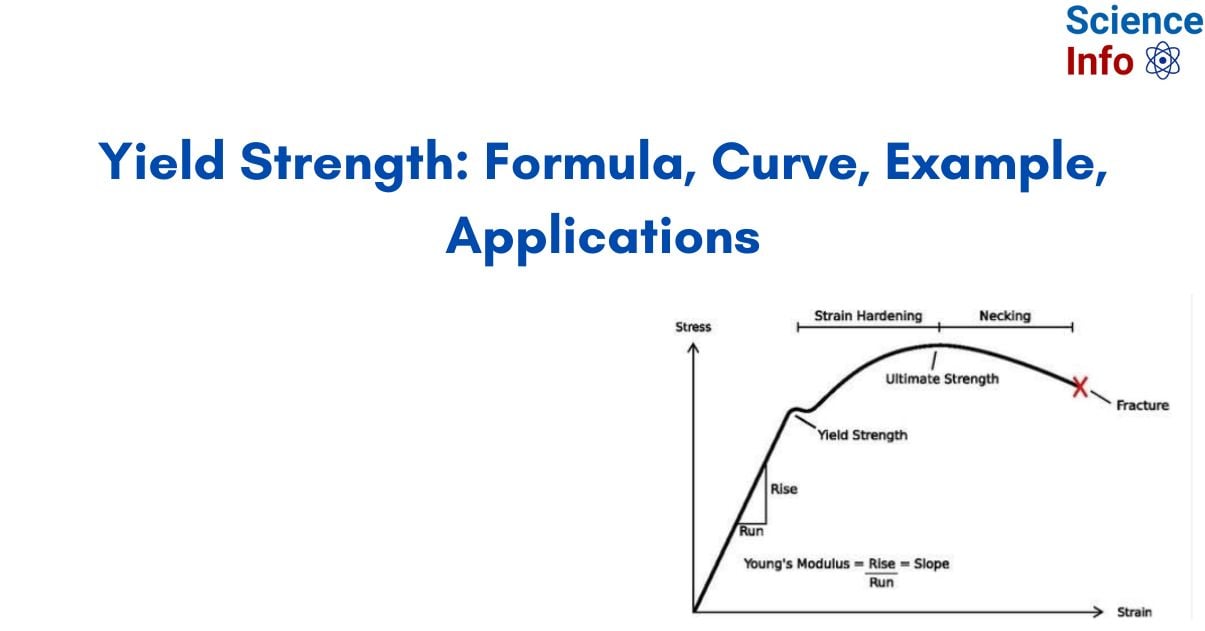
Interesting Science Videos
What is Yield Strength?
- Yield strength is the highest stress to which a material can be subjected before experiencing plastic deformation. When referring to ductile, or deformable, materials, the phrase “yield strength” is usually employed.
- σY is the yield strength symbol. The subscript “Y” stands for “yield,” while the Greek letter σ is the symbol for engineering stress. “SY” is also occasionally used to indicate yield strength.
- The SI unit of stress, Pascals (Pa), or pounds per square inch (psi) are commonly used to express yield strength.
- It designates the location on the stress-strain curve at where the material changes from elastic deformation, which occurs when the load is removed, to plastic deformation, which occurs when the material maintains a permanent shape change.
- Tensile testing, in which a material specimen is subjected to increasing tensile forces until it reaches the point of yielding, is commonly used to assess yield strength. Known as the yield point or yield stress, this is distinguished by a clear departure from the linear elastic area of the stress-strain curve.
- Because it shows the highest stress a material can bear before permanently deforming, yield strength is an essential property in materials engineering. Given that it controls when yielding will begin and when a structure may break under stress, it is very significant in structural and mechanical design. The yield strength is used by engineers to design structures and parts with the proper safety margins to avoid plastic deformation while they are in operation.
- The reason yield strength matters is that it represents the maximum stress a material can withstand before experiencing irreversible deformation. When determining the maximum load that a structure or part built for it can support, engineers frequently employ the yield stress of a material. Engineers can create parts that are more robust and safe by using knowledge of a material’s yield strength.
How to Measure Yield Strength?
Tensile testing is the most widely used method for measuring yield strength, which is a crucial mechanical attribute of materials. Following are the steps to measure yield strength:
- Standardized test specimens must be prepared in accordance with relevant standards or specifications as the first stage. These specimens often have precise measurements and a cylindrical or rectangular shape. The test findings are certain to be consistent and uniform due to meticulous preparation.
- A tensile testing machine is used to mount the prepared specimen and apply a regulated tensile force on it. The apparatus consists of load cells to precisely measure the applied force and grips to hold the specimen firmly.
- The test begins with the application of a consistent axial load, as stated by standards such as ASTM or ISO, to the specimen. The specimen first experiences elastic deformation as the load is gradually raised.
- The apparatus measures the specimen’s elongation as a result of the applied stress and records it simultaneously during the test. The specimen’s stress (force per unit area) and strain (deformation) are calculated using these values.
- The material deforms elastically and returns to its original shape upon unloading, and this is represented by the linear elastic region that the stress-strain curve first follows as the load increases. The curve begins to diverge from linearity at a certain point, signifying the start of plastic deformation. This deviation represents the yield point, also known as the yield stress, which denotes the start of the material’s permanent deformation.
- Depending on the material behavior and testing requirements, there are various approaches for calculating yield strength. Typical methods involve finding the point of intersection between the stress-strain curve and a certain yield criterion, determining an offset from the linear portion of the stress-strain curve (e.g., 0.1% or 0.2% offset yield strength), and applying the proportional limit.
- Engineers examine the data collected from the test to ascertain the material’s yield strength. This entails analyzing the stress-strain curve, locating the yield point or yield stress, and applying the selected method to determine the related yield strength.
- Along with other significant mechanical properties, test parameters, and specimen data, the measured yield strength is presented. For the purposes of design, quality assurance, and material characterisation, this data is crucial.
Yield Strength Formula
The basic method for calculating stress is force divided by area normal to the force, and this is the mathematical formula for yield strength, or stress at yielding. The applied force at the beginning of plastic deformation divided by the test sample’s initial cross-sectional area is the precise definition of yield strength. The following is the formula for calculating yield strength:
Yield Strength=σy=Sy=F/A
Engineering stresses at the lower yield point or at 0.2% offset strain can be used to determine the stress that is used to compute yield strength.
Explanation of Stress-Strain Curve
The following are the various points or zones on the stress-strain graph:
- Proportional limit: Hooke’s Law is followed in the stress-strain curve region. The stress-to-strain ratio in this limit provides us with a proportionality constant called Young’s modulus. The proportional limit is the point OA on the graph.
- Elastic limit: It is the location on the graph where, in the absence of any load, the material reverts to its initial state. Beyond this point, the material begins to exhibit plastic deformation and fails to return to its initial shape.
- Yield point: The point at which a material begins to deform plastically is known as the yield point. Plastic deformation becomes irreversible once the yield point is reached. The term “yield point” refers to the upper and lower yield points, respectively.
- Ultimate stress point: This point denotes the highest stress a material can withstand prior to failing. Beyond this moment, something fails.
- Fracture or Breaking point: It represents the location on the stress-strain curve where the material fails.
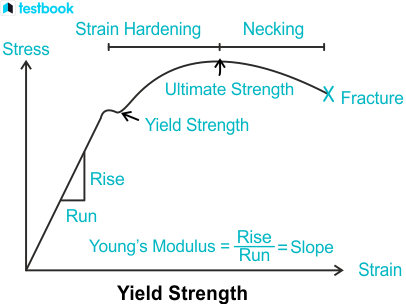
It can be described comprehensively as:
- Yield point: The observed point on the stress-strain curve when plastic deformation starts is known as the yield point. Between the onset of the tensile stress and the yield point, the material undergoes elastic deformation. By determining the boundaries of the non-linear and linear portions of the stress-strain graph, one can predict the yield point. Mild steel is one of the metals with two yield points.
- Elastic limit: The point on the stress-strain curve known as the elastic limit is the highest strain that a material can sustain before permanently deforming. The material reverts to its initial proportions upon cessation of tension. There is no more distortion after the elastic limit.
- Proportionality limit: The point where the linear section of the stress-strain curve ends is known as the proportionality limit. Stress and strain in the material are directly proportional up to the proportionality limit. The material’s Young’s modulus, often known as its modulus of elasticity, is the ratio of stress to strain. Stress and strain no longer have a linear relationship after the proportionality limit is exceeded.
- True elastic limit: The lowest stress at which dislocations in a material’s crystalline structure can move is known as the true elastic limit. Since dislocations can move at low stress and are difficult to detect, it is rarely utilized to define material yield.
- Upper and lower end points: Because of the fast formation of dislocations in the crystal lattice, the upper yield point marks the beginning of plastic deformation in the test specimen. This point, however, is unstable and not a useful starting point for design work because it depends on test equipment and strain rate. The lower yield point designates a time interval prior to the onset of strain hardening, during which localized plastic deformation bands, also known as Luders bands, form and expand at nearly constant tension across the test section. It is more conservative and more reproducible to use the lower yield point.
- Offset Yield Stress: The most popular way to describe the yield strength of a material is to use the offset yield stress, often known as the proof stress. Drawing a line parallel to the linear section of the stress-strain curve yields the answer. There is a 0.2% positive strain offset in this line. The material’s yield strength is determined by taking the point on the stress-strain curve where this offset line meets it.
Interpretation of Stress-Strain Curve Graph of Yield Strength
One can understand yield strength by examining a material’s stress-strain curve.
- Determine which area of the graph is linear first. This part of the curve represents the relationship between stress and strain.
- Second, determine the point on the curve where the non-linear component starts and the linear portion finishes.
- A material’s yield strength can be determined in a number of ways using its stress-strain graph. The most widely used method for describing the yield strength of a material is known as the “0.2% offset yield strength.” A line is formed on the stress-strain curve parallel to the initial linear, elastic stress-strain loading curve in order to calculate the 0.2% offset yield strength. This offset line’s origin is at 0.2% strain (0.2% on the x-axis) and zero stress (0 on the y-axis).
- The material’s yield strength is determined by finding the point on the actual stress-strain curve where this offset parallel line intersects it.
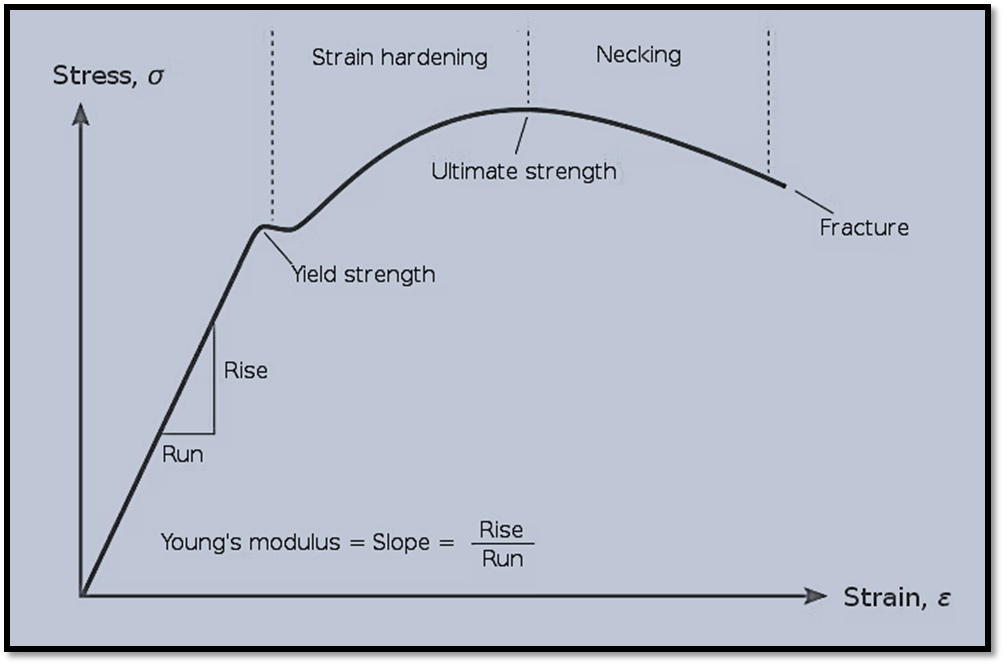
Examples of Yield Strength
A material’s chemical composition, crystal structure, and whether or not it is a fiber-reinforced composite all affect its yield strength. Below is a list of several sample materials’ yield strengths:
- Steels: Hot-rolled A36 steel has a yield strength as low as 220 MPa, whereas oil-quenched and tempered 4140 alloys have a yield strength as high as 1570 MPa.
- Stainless Steel: Austenitic stainless steel has a yield strength of approximately 250 MPa, while precipitation-hardened stainless steel has a yield strength of 1000 MPa.
- Alloys made of aluminum: The yield strengths of these materials vary from 24 MPa (1100 aluminum alloy) to 483 MPa (7075 aluminum alloy).
- Plastics: Plastics have yield strengths ranging from 4 MPa (plasticized PVC) to 300 MPa (PA 66 packed with carbon fiber).
Application of Yield Strength
- In structural engineering, where materials are subjected to pressures and stresses in buildings, bridges, dams, and other infrastructure projects, yield strength is vital. In order to assure that structural elements like beams, columns, and trusses can handle expected loads without experiencing severe plastic deformation, engineers use yield strength in their design process. Engineers can design structures that minimize weight and cost while meeting safety norms and performance criteria by using materials with the right yield strengths.
- Yield strength affects material behavior and processing parameters in metal forming, welding, and machining, among other production processes. When creating forming dies, choosing welding techniques, and setting machining parameters, manufacturers need to take the yield strength of the materials into account in order to avoid flaws like distortion, excessive springback, and cracking. Gaining an understanding of yield strength aids in streamlining production procedures and preserving the integrity and caliber of assembled parts.
- In many different industries, the selection and design of materials heavily relies on yield strength. When selecting materials for certain applications, engineers take into account yield strength in addition to other mechanical characteristics like tensile strength, ductility, and toughness. For instance, materials with sufficient yield strength are chosen for consumer goods, packaging, and electronics so they can endure production procedures, shipping, and service conditions without breaking down or deforming. Through material selection that either matches or surpasses expected yield pressures, engineers guarantee the dependability, durability, and contentment of their products.
- In aircraft and automotive applications, where lightweight materials with high strength are necessary for performance, safety, and fuel efficiency, yield strength is crucial. For vehicles such as cars, spaceships, and airplanes, materials with high yield strengths are used to minimize weight and maintain structural integrity and crashworthiness. Examples of these materials are advanced high-strength steels, aluminum alloys, and titanium alloys. To achieve strict performance and safety regulations, engineers optimize the yield strength-to-weight ratio of materials used in the construction of components such as fuselage frames, engine mounts, and chassis structures.
- In sectors including building, mining, and oil & gas, yield strength is crucial for guaranteeing safety and legal compliance. To avoid accidents, environmental risks, and structural collapses, regulatory bodies and standards organizations set minimum yield strength limits for materials used in crucial applications. To confirm compliance and guarantee the integrity and dependability of engineered systems, engineers must follow these specifications and carry out stringent materials testing and quality control.
References
- https://unacademy.com/content/jee /study-material/physics/yield-strength/
- https://testbook.com/physics/yield-strength
- https://www.toppr.com/guides/physics/elasticity/yield-strength/
- https://www.xometry.com/resources/3d-printing/yield-strength/#:~:text=What%20Is%20Yield%20Strength%3F,or%20materials%20that%20can%20deform.
- https://byjus.com/physics/yield-strength/