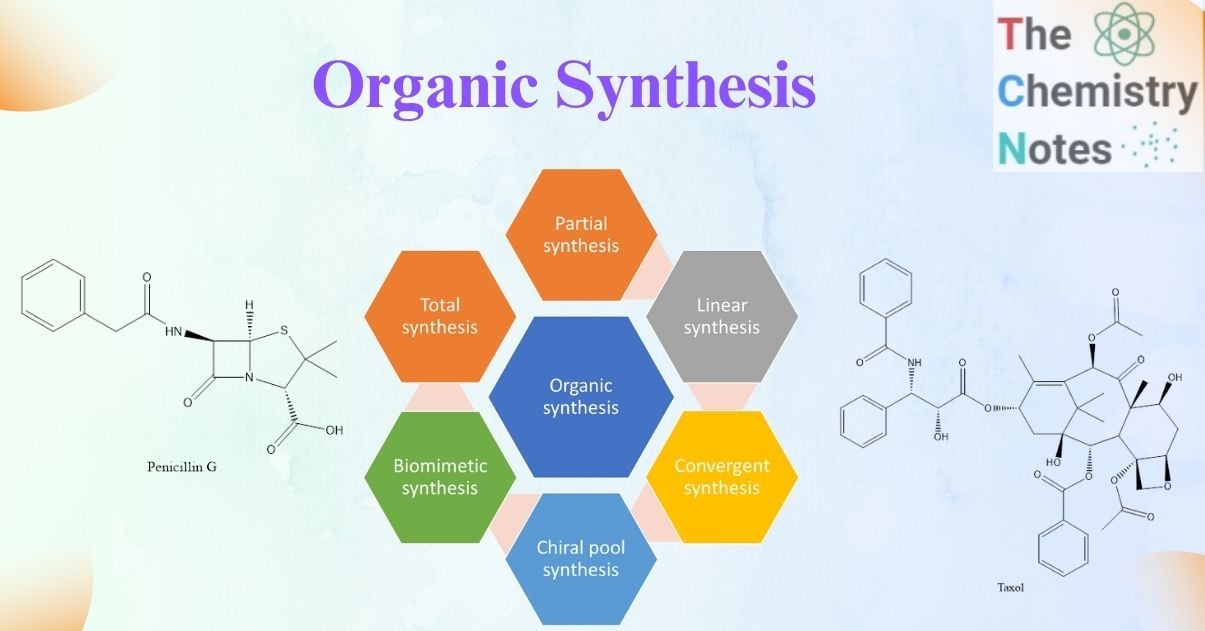
Organic synthesis involves a specific series of chemical reactions that transform starting elements into the desired product, known as the synthesis target (or target molecule). Synthesis is often the result of numerous distinct processes known as synthetic stages.
Interesting Science Videos
History of organic synthesis
Since Friedrich Wohler’s unintentional manufacture of urea almost two centuries ago, organic synthesis, a discipline of chemistry that comprises the preparation of organic molecules by a sequence of well-orchestrated chemical manipulations, has seen over two hundred years of innovation. The early days of synthetic organic chemistry, like many other sciences, were full of unanticipated shocks. Wohler’s urea synthesis and Perkin’s mauveine synthesis were two of the most renowned examples of unintentional discoveries made based on elemental compositions alone, in the lack of even the most elementary structure and bonding ideas.
Chemists’ fascination with molecular complexity brought in the next era of organic synthesis, and while there were many notable contributors at the time, this golden period of organic synthesis was best captured by the late Professor Robert Burns Woodward’s dominance, and became known as the “Woodwardian” era. The interaction of physical approaches and synthetic chemistry also permitted structural interrogation and validation of chemical entities with considerably better efficiency and accuracy.
Although the increasing sophistication of organic synthesis in the Woodwardian era was well-appreciated at the time, this academically hard discipline relied greatly on the practitioner’s insight and experience. Indeed, it was not long before Professor Elias J. Corey conceptualized retrosynthetic analysis, ushering in the next breakthrough phase of organic synthesis.
Woodward’s creativity and vision, along with Corey’s retrosynthetic logic and rigor, lifted synthetic organic chemistry to new heights in the late twentieth century.
Strategies of organic synthesis
There are various methods for synthesizing organic molecules. The main strategies involve:
I. Formation of the carbon-carbon bond.
II. Function group based synthesis strategy
III. Selection-based synthesis strategy
IV. Symmetry-based synthesis strategy
Formation of the carbon-carbon bond
I. Formation of C – C bond
Different organic synthesis reaction involves the formation of a bond between the two carbon atom of reacting molecule. Some examples are:
Claisen condensation
The Claisen condensation process is an organic coupling reaction that produces a C-C bond between two molecules of ester to give beta-keto ester. In this reaction, esters with α hydrogen undergo a condensation reaction, in the presence of a strong base (C2H5ONa), to form β keto ester.

In the presence of dry ether, the Grignard reagent combines with carbonyl compounds to produce an additional product that, upon acidic hydrolysis, yields the corresponding alcohol. This is an example of a nucleophilic addition reaction. Thus formed alcohols can be 1o, 2o, or 3o depending on the type of carbonyl compounds utilized.
The addition of Grignard reagents to formaldehyde results in the formation of primary alcohols. Secondary alcohol is produced when the Grignard reagent is reacted with an aldehyde other than formaldehyde.
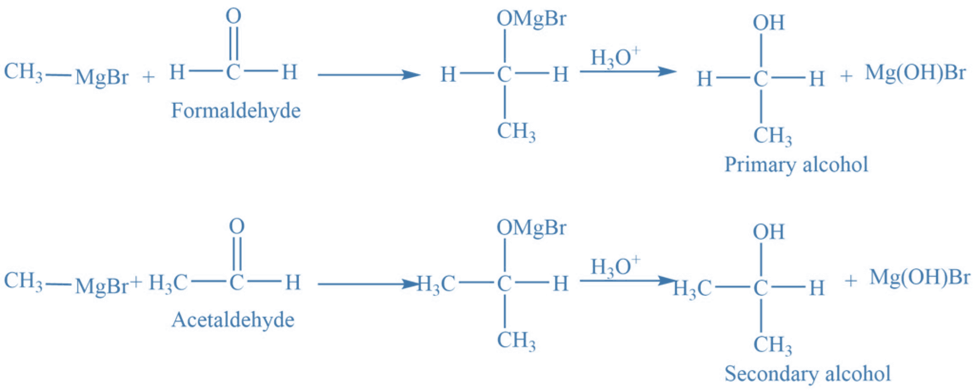
Diels alder reaction
This reaction involves the reaction between two unsaturated compounds. In this reaction, a dienophile reacts with a diene to give cyclocompound. This reaction can take place in the presence or absence of a solvent, and it can be initiated by light, heat, or AlCl3 as a catalyst.
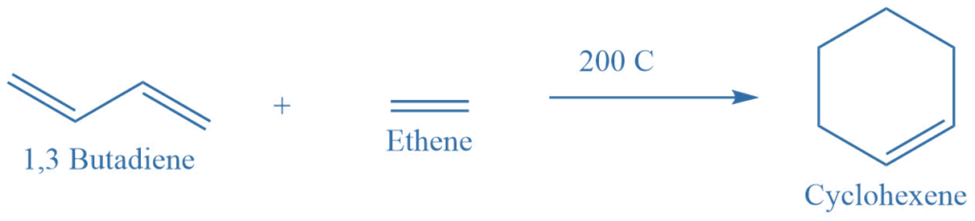
Formation of C = C bond
Wittig reaction
The Wittig reaction is an organic chemical reaction that involves the reaction of aldehyde or ketone with a triphenyl phosphonium ylide ( Wittig Reagent) to produce an alkene and triphenylphosphine oxide and triphenylphosphine oxide.

Elimination reaction
Elimination reactions involve the removal of a small molecule from a larger one. Elimination reactions generally produce C = C bond. For example: For example, 2-Bromo-2- methyl propene in reaction with aqueous ethanol at 25oC produces 2- Methyl propene.
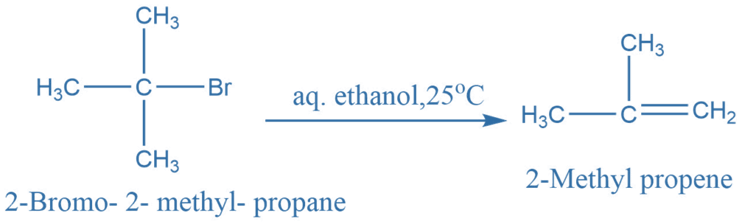
It is an example of an unimolecular elimination reaction.
Aldol condensations
Aldol condensations are important in organic synthesis reactions because they provide a consistent technique for generating carbon-carbon bonds. Two aldehyde or ketone molecules having at least one hydrogen atom undergo a condensation reaction in the presence of a diluted alkali, to produce β-hydroxy aldehydes or aldol and β-hydroxy ketones or ketol. Which removes the water molecule to give α– β unsaturated product.
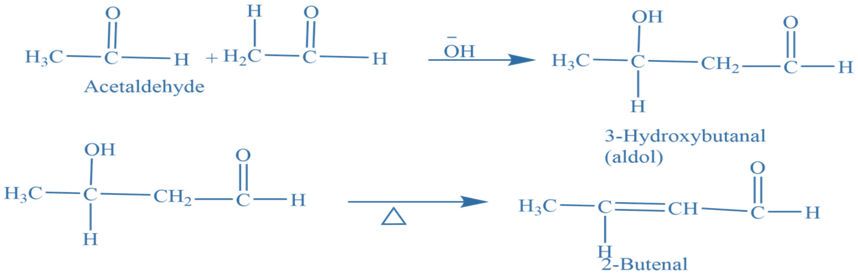
Function group strategy
Function group strategy involves an introduction, deletion, and function group modification or alteration of functional groups in order to obtain the desired compound’s functionality.
Function group modification
oxidation
Baeyer villager oxidation
It entails oxidizing aliphatic ketones to esters or their hydrolyzed products with hydrogen peroxide or organic peracids. The oxidative transformation of an aldehyde and ketone into carboxylic ester and cyclic ketone to lactone utilizing peroxy substances such as hydrogen peroxide and peroxy acids in the presence of an acidic catalyst is known as Baeyer villager oxidation.
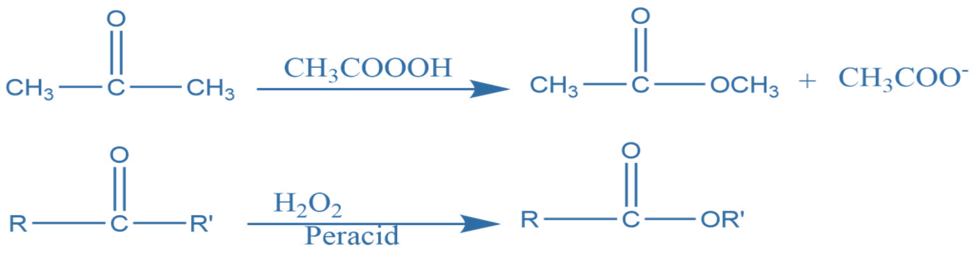
Oppenauer oxidation is a chemoselective oxidation reaction in which secondary alcohols are converted to ketones in the presence of aluminum tertiary butoxide in benzene or toluene solution. Aluminum alkoxide catalyzes the oxidation of a secondary alcohol to the corresponding ketone. To accelerate the reaction forward in the desired direction, a substantial excess of the ketone is utilized.

Chromic acid oxidation
Chromic acid is commonly employed for the oxidation of alcohols, aldehydes, and hydrocarbon carbon-hydrogen bonds. Typically, chromic acid is produced in situ by adding acid to a chromium source. Sodium dichromate, potassium dichromate, sodium chromate, potassium chromate, and chromium trioxide are major chromium sources and oxidizing agents.
For example, When an alcohol solution in acetone is treated with the Jones reagent (a solution of chromic acid and sulfuric acid in water), it yields ketone without disrupting any double or triple bonds that may be present.
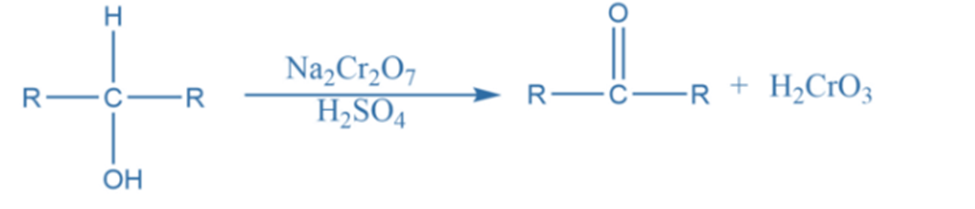
Catalytic hydrogenation
The direct addition of molecular hydrogen to a double bond in the presence of a transition metal catalyst such as Ni, Pt, Pd, Ru, or their derivatives is known as catalytic hydrogenation.
The reaction is thought to involve chemisorption, which is the exothermic adsorption of gases by solids that involves the development of a chemical bond between the adsorbing and adsorbed components.
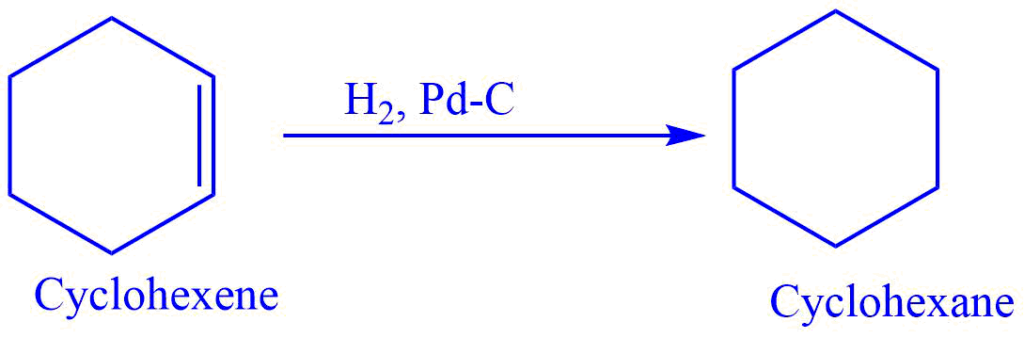
Clemmensen reduction
In the presence of concentrated hydrochloric acid, aldehyde or ketone interacts with Zn-Hg to produce the corresponding hydrocarbon. Clemmensen reduction is the name given to this reaction.
The acid in this reaction provides a proton to the oxygen. At the same time, metal provides an electron pair to the electron-deficient carbonyl carbon, resulting in carbanion formation.
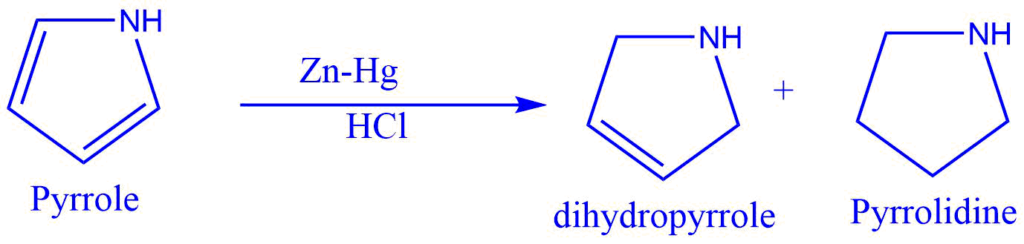
Metal hydride reduction
The conversion of aldehyde and ketone into corresponding primary and secondary alcohols in the presence of metal hydrides such as lithium aluminum hydride (LiAlH4) or sodium borohydride (NaBH4) is known as metal hydride reduction.
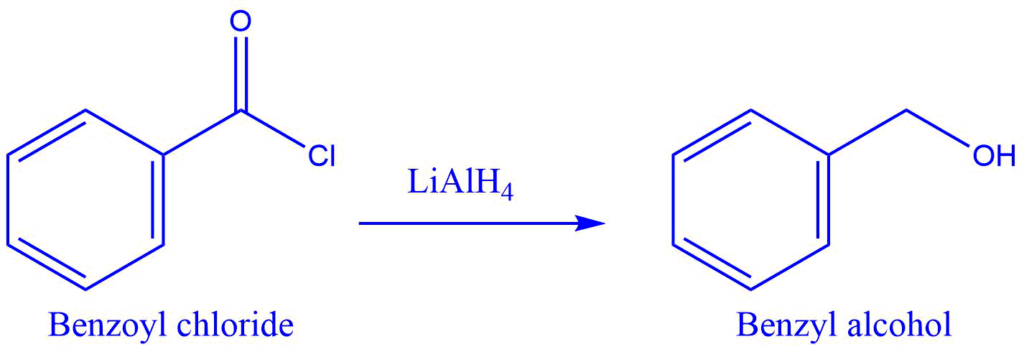
Insertion of functional group
Halogenation of alkane
Halogenation of alkanes is the process of replacing one or more hydrogen atoms in organic compounds with halogen atoms (such as F, Cl, Br, I). The halogenation of alkanes follows a free radical mechanism.
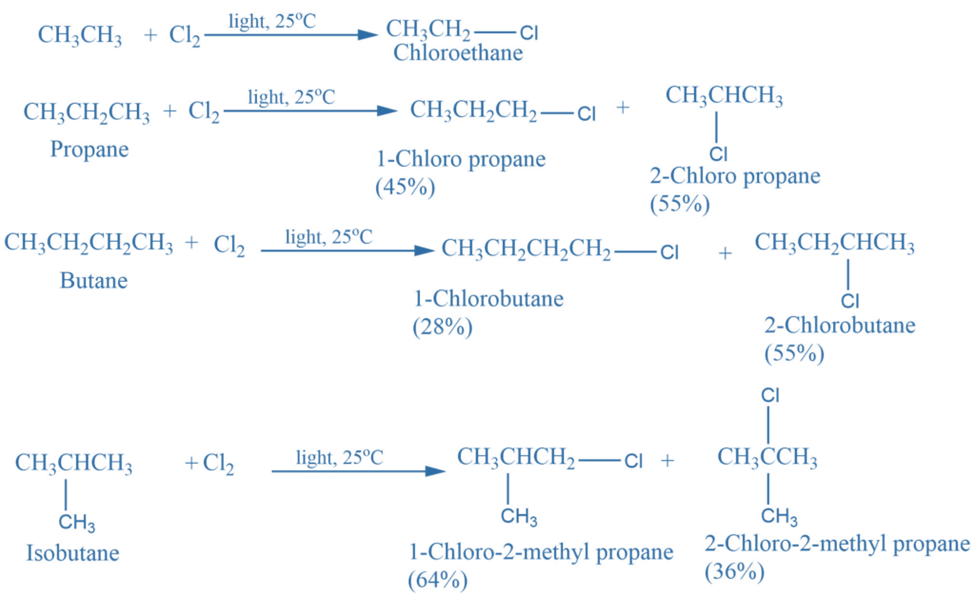
Nitration reaction
Nitroalkane is produced by heating alkane with nitric acid fumes at 400-500°C. The procedure is known as Vapour Phase Nitration.

Sulphonation reaction
When benzene reacts with concentrated sulfuric acid, it produces benzene sulphonic acid, which when reacted with sodium hydroxide or sodium sulfite yields sodium sulphonate. Sodium phenoxide is formed by combining sodium sulphonate with caustic soda. When this product is hydrolyzed and subsequently acidified with SO2 or HCl, it generates phenol.
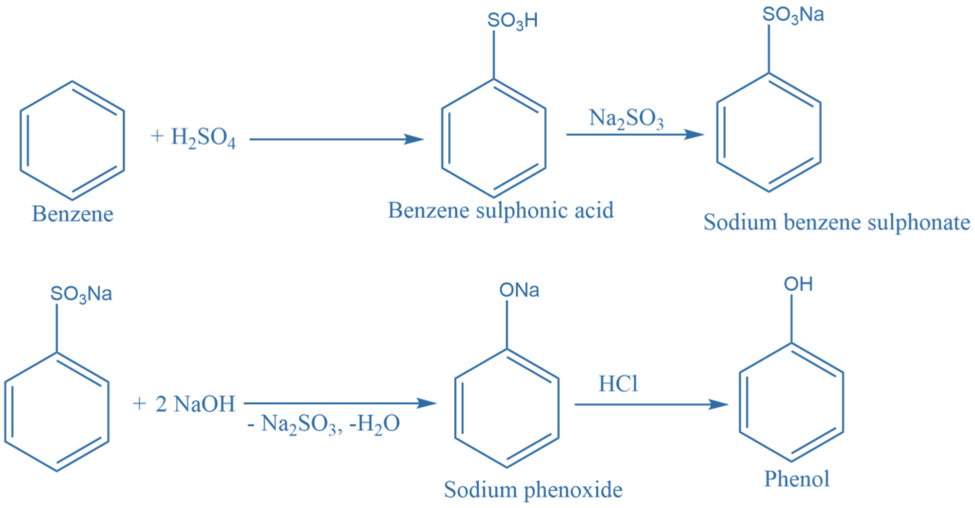
Removal of functional group
Decarboxylation reaction
Dieckmann’s method, which is an intermolecular claisen condensation reaction, provides an important strategy for the synthesis of five, six, and seven member alicyclic compounds.
The ester of a suitable carboxylic acid undergoes cyclization upon interaction with sodium metal or sodium ethoxide, yielding 2-carboalkoxycycloalkanone, which upon hydrolysis generates 2-carboxycycloalkanone. Further decarboxylation of 2-carboxycycloalkanone produces cycloalkanone, which is reduced to form cycloalkane.
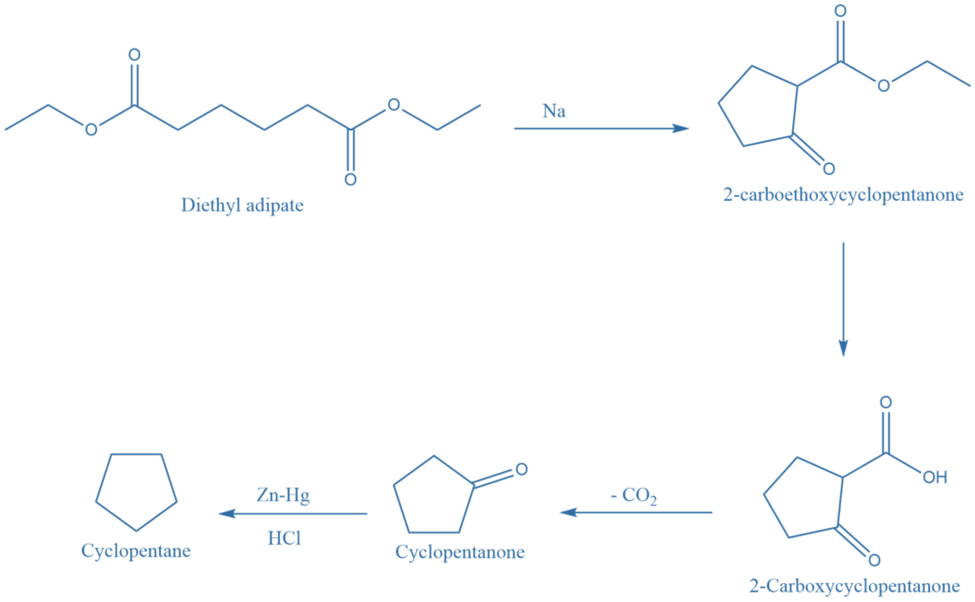
Dehydrohalogenation reaction
When alkyl halide is heated with alcoholic potassium hydroxide, an alkene is formed as a major product while a hydrogen halide is generated as a byproduct. This reaction follows the Saytzeff rule.
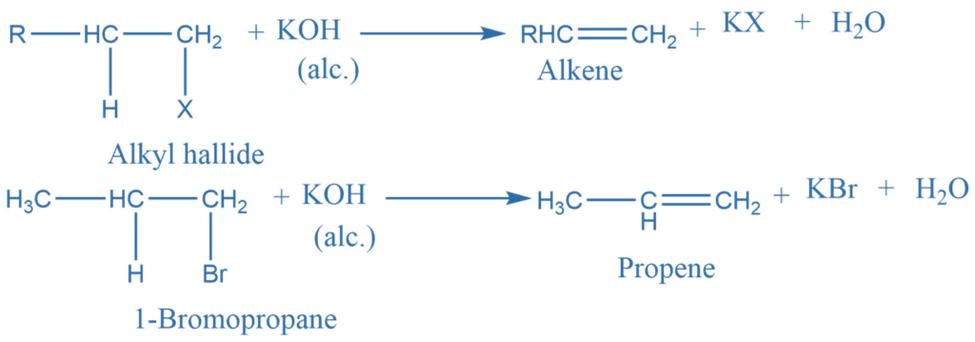
Dehalogenation reaction
Terminal dihalides undergo cyclization reactions when exposed to salt or zinc, yielding cycloalkanes. Terminal halogen derivatives with hydrogen positions other than the 1-6 position undergo the Wurtz reaction rather than the cyclization reaction.

Selectivity based synthesis
Regioselective synthesis
Regioselective synthesis reactions are chemical reactions in which one reaction site is favored over another in a chemical reaction. e.g., Hydroboronation oxidation reaction.
The hydroboration-oxidation reaction, in which the hydroxyl group attaches to the less-substituted carbon, is an anti-Markovnikov reaction. Hydroboration is a highly selective and stereospecific reaction. Borane addition is characterized by steric and electrical phenomena that favor the attack over the less substituted carbon. Boron selects the least substituted carbon to avoid steric hindrance while adding the second and third alkene residues.
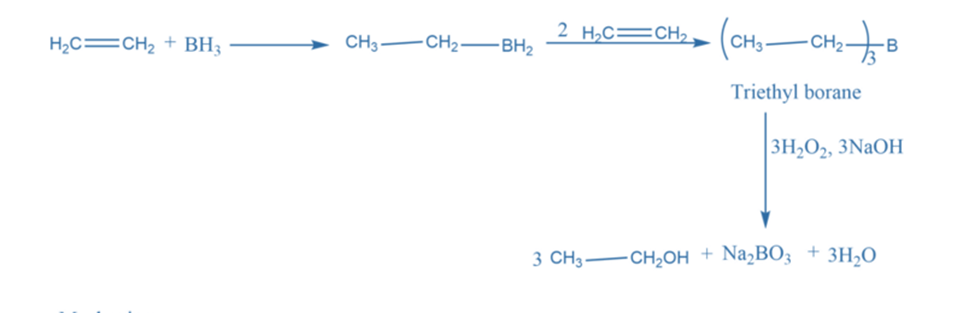
Chemoselective synthesis
Chemoselectivity is the selective reactivity of one functional group in the presence of others. It is the preferential interaction of a chemical reagent with one or more functional groups. If only a few distinct functional groups react with a reagent, it has a high chemoselectivity. Sodium tetrahydroborate, for example, is a more chemoselective reducing agent than lithium tetrahydro aluminate.
Chemoselective C=C reduction over C=O:
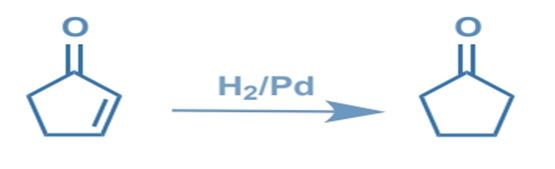
Symmetry based synthesis
Asymmetric (Stereoselective) synthesis
An asymmetric reaction is a chemical reaction (or reaction sequence) in which one or more additional components of chirality are produced in a substrate molecule and which creates stereoisomeric (enantiomeric or diastereoisomeric) products in uneven proportions. They are historically known as asymmetric synthesis.
It means having some degree of stereochemical control. More specifically, it refers to control over new stereochemistry. Stereospecificity is a reaction mechanism attribute that results in multiple stereoisomeric reaction products from separate stereoisomeric reactants. A stereoselective process is one in which one stereoisomer predominates over another when two or more are possible.
Enantioselective synthesis
When one of the enantiomeric products of a chemical process predominates, the reaction is referred to as enantioselective synthesis. Although the substrate molecule usually controls diastereoselective synthesis, enantioselective reactions occur under the control of the reagent.
E.g. Monoisopinocamphylborane (IPC)BH2 is a very good enantioselective reagent for hydroboration of trans alkene, trisubstituted alkene, and 1- substituted alkene.
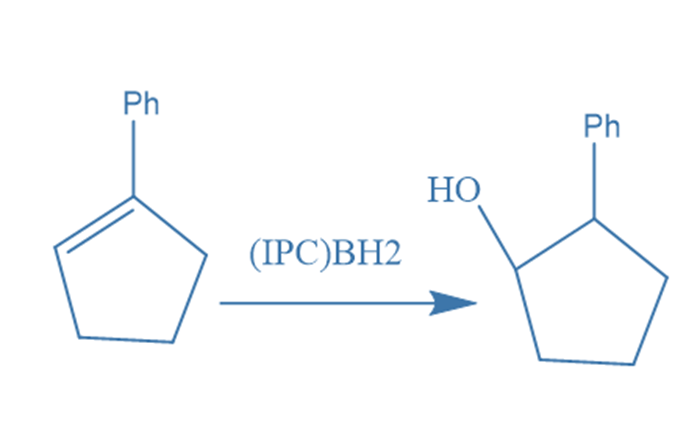
Diastereoselective synthesis
When one diastereomer predominates over the other, the reaction is referred to as a diastereoselective reaction. Generally, new chiral centers are created on the chiral substrate molecule during diastereoselective synthesis. The insertion of groups on diastereotopic faces of double bonds is the most prevalent method of creating new chiral centers.
Chirol pool synthesis
A chiral pool is a group of naturally occurring enantiomerically pure compounds.
Amino acids, chiral carboxylic acids, and monosaccharides are common natural chiral starting materials. It is one of the simplest and oldest methods of enantioselective synthesis. In this synthesis, an enantiomerically pure natural substance is used as a starting material.
The chiral pool—Nature’s’ready-made chiral centers: pure natural compounds, typically amino acids or sugars, from which pieces containing the requisite chiral centers can be extracted and incorporated into the product.
A Chiron method, also known as chiral pool synthesis, is a synthetic procedure that uses a chiral pool member as a starting material (SM) in the synthesis of a target molecule (TM). The chiral center (s) of the beginning material is conserved (but not always) in the target molecule (TM). It could influence the production of new chiral centers by using pre-existing chiral centers from the chiral pool substrate. Substitution or addition reactions can be used to create new chiral centers.
e..g., Chiral pool synthesis of the essential amino acid, L-lysine from the natural non-essential amino acid L-serine
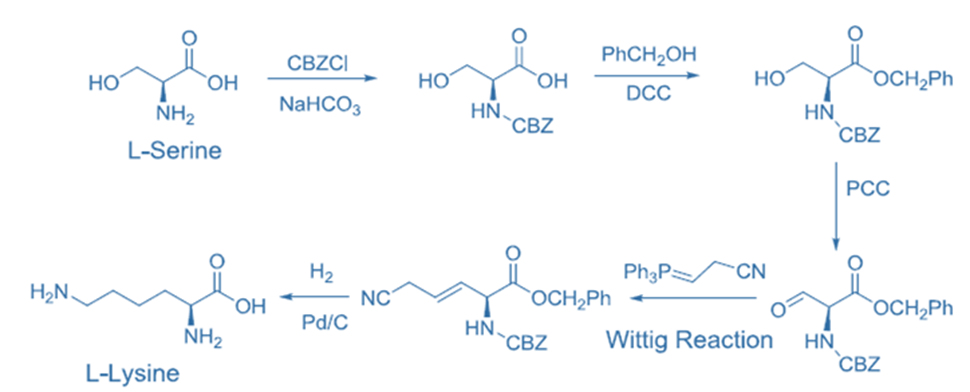
Types of organic synthesis
Linear synthesis
Organic synthesis, in which the target molecule is generated from the starting molecule in a stepwise manner, is called linear synthesis. A linear pathway is one in which all reagents are added sequentially.
A (starting molecule) → B → C → D → D (target molecule)
e.g., Synthesis of carboxylic acid from ethane through various chemical steps:
CH3CH3 → CH3 CHO → CH3 COOH
As linear synthesis involves the formation of the product via various steps, the overall yield decreases rapidly with each reaction step. e,g,

Since the overall yield of the synthesis is based on the single longest route to the target molecule, a linear synthesis has a lower overall yield. Because of its lack of flexibility, linear synthesis is susceptible to failure, potentially resulting in huge losses in material already invested in the synthesis at the time of failure. It is time consuming and rigorous method.
Convergent synthesis
In a convergent synthesis, components of a target molecule are synthesized separately and then joined together at a later step. Therefore, in this synthesis process, major components of the target molecule are synthesized separately or independently and then brought together at a later point in the synthesis to form the target molecule.

A convergent synthesis is more efficient and shorter than a linear synthesis, resulting in a greater total yield. Because of the independent synthesis of the target molecule fragments, it is more adaptable and easier to carry out.
Divergent synthesis
A divergent synthesis is a different approach that begins with a molecule as a central core and adds subsequent generations of building blocks to it. A divergent synthesis is a chemistry approach used to increase the efficiency of chemical synthesis. Divergent synthesis is a technique that involves first reacting a molecule with a group of reactants in order to produce a library of chemical compounds.
Divergent synthesis, as opposed to target-oriented synthesis, includes the planned synthesis of a succession of chemicals from a single intermediate. This method is especially useful when the subsequent applications include high-throughput screening processes. Many biomacromolecules, such as DNA and proteins, are also generated in a diverse manner, either spontaneously or chemically, from a finite set of nucleotides and amino acids, respectively.
The primary problem for divergent synthesis is that the designed route be clear and generally robust in order to prepare the diverse targets in an efficient, simultaneous, and combinatorial manner.
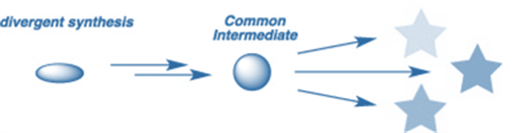
Divergent synthesis is an approach for increasing the efficiency of chemical synthesis. It is frequently used as an alternative to convergent or linear synthesis. Divergent synthesis tries to produce a chemical compound by first reacting a
molecule with several reactants. This process rapidly leads to a synthesis of a number of new molecules.
Total synthesis
The technique of synthesizing organic compounds using small and inexpensive readily available molecules derived from bio reactants and then reacting them to produce a product.
Total synthesis begins with a tiny organic molecule and proceeds through a series of reactions to make a product. Examples of cholesterol synthesis
Partial synthesis
Partial synthesis is the process by which compounds isolated from natural sources (plant material, coal, or petroleum) are used as starting materials for producing other Organic compounds. The Synthetic technique converts them into hundreds of valuable chemicals.
Examples: Carbohydrates (Starch, Cellulose)
Biomimetic synthesis
” Biomimetic synthesis is a branch of natural product synthesis that seeks to synthesize a target molecule via a series of reactions and intermediate structures that are closely similar to those observed during biosynthesis in its natural source. Sir Robert Robinson, an English organic scientist, invented the term “biomimetic synthesis” in 1917. He was awarded the Nobel Prize in 1947 for his work on plant dyestuffs and alkaloids.
Robinson synthesized tropinone, a bicyclic compound, from succinaldehyde, methylamine, and acetonedicarboxylic acid.
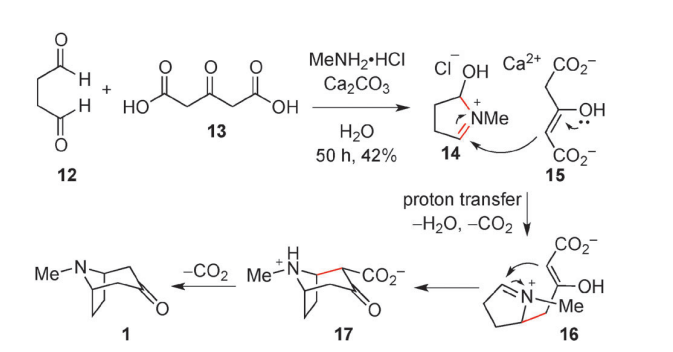
Robinson’s tropinone synthesis
Image source: https://doi.org/10.1039/C3CC44461A
In this reaction process, Succindialdehyde (12) reacts with Acetone dicarboxylic acid(13) to give Tropinone (1). The reaction proceeds through the formation of Cyclic iminium (14) and other intermediates (16), (17).
Robinson’s tropinone synthesis is a notable example of biomimetic synthesis. Because of its simplicity and biomimetic approach, Robinson’s synthesis is regarded as a classic in total synthesis.
Biomimetic synthesis is commonly used to describe syntheses that mimic biological synthesis as it occurs in live organisms. Biomimetic synthesis is the testing of a “biosynthetic hypothesis” (the supposed process of biosynthesis in nature) through a sequence of reactions that intends to parallel the hypothesized biosynthesis and research programs in which synthetic reactions meet their goals.
Retrosynthesis
E. J. Corey put forward a retrosynthetic strategy in which the target structure is subjected to a deconstruction process, which corresponds to the inverse of a synthetic reaction. Retrosynthesis is the process of “deconstructing” a target molecule into readily available starting materials by means of the imaginary breaking of bonds and by the conversion of one functional group into another (functional group interconversions).
It’s useful for finding out novel methods for the synthesis of new pharmaceuticals or existing drugs and comparing them in a logical & straightforward approach. It is the process of working backward from the target molecule in order to devise an acceptable synthetic pathway. In this technique, molecules are intensionally broken to find the starting material.
Retrosynthetic analysis is based on known reactions (e.g. the Wittig reaction, oxidation, reduction, etc). The synthetic plan generated from the retrosynthetic study will be a guide to direct the synthesis of the target molecule. So, retrosynthetic analysis (retrosynthesis) is a technique for planning synthesis, particularly of complex organic molecules, in which the complex target molecule (TM) is reduced into a series of progressively simpler structures along a pathway, eventually leading to the identification of a simple or commercially available starting material (SM) from which a chemical synthesis can be developed.
TM → SM →Starting material
E.g., Retrosynthesis of Paracetamol
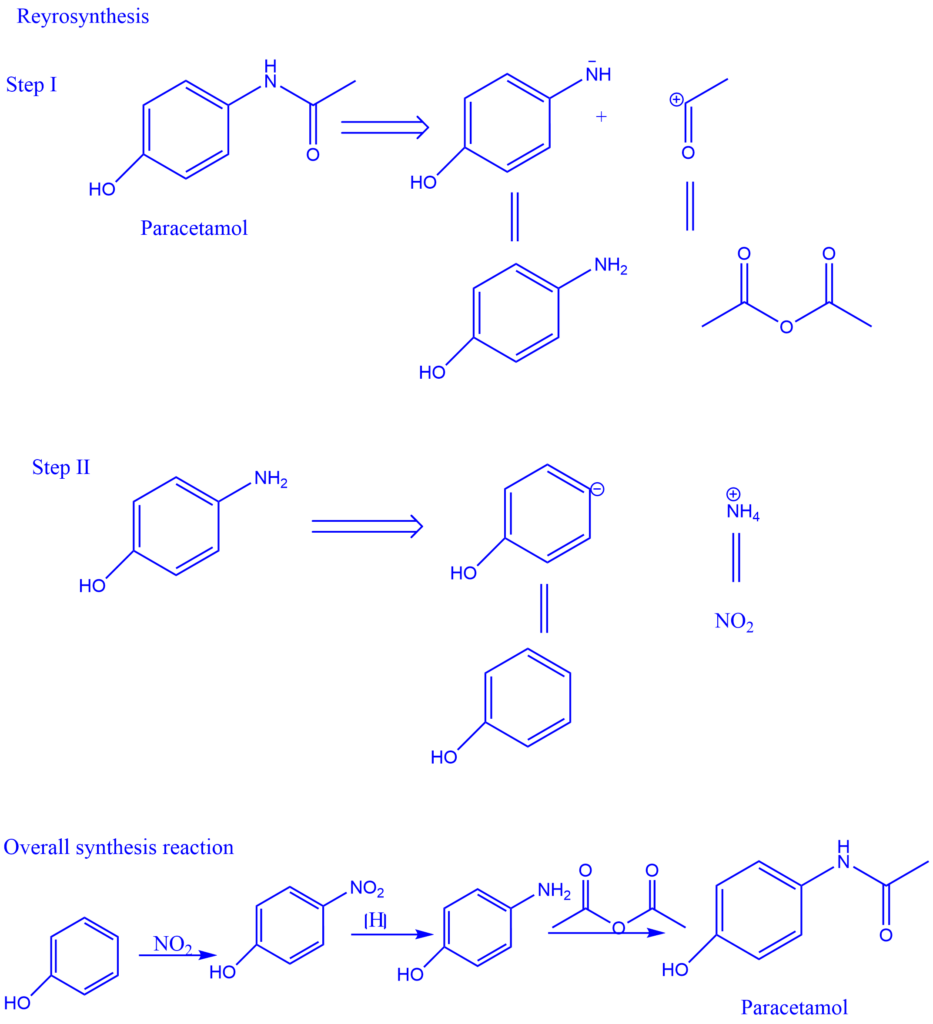
Combinatorial synthesis
Combinatorial synthesis is a method of synthesizing a large number of compounds in a short period of time by employing a predetermined reaction route and a wide range of starting materials and reagents. Combinatorial synthesis employs procedures and strategies, which enable the quick formation of large, structured collections of organic molecules, known as molecular libraries.
The combinatorial approach can synthesize millions of compounds in the time it takes to produce one product using the conventional approach of synthesis. However, the efficiency of a molecule is heavily influenced by its size, solubility, and function group. Compounds generated are typically Achiral or Racemic.
References
- Smith M. & March J. (2001). March’s advanced organic chemistry: reactions mechanisms and structure (5th ed.). Wiley.
- Organic-synthesis-strategy-and-control-by-stuart-warren11.
- https://www2.chemistry.msu.edu/faculty/reusch/virttxtjml/synth2.htm.
- https://diposit.ub.edu/dspace/bitstream/2445/364/1/104.pdf.
- https://chem.libretexts.org/Courses/Athabasca_University/Chemistry_350%3A_Organic_Chemistry_I/09%3A_Alkynes_An_Introduction_to_Organic_Synthesis/9.09%3A_An_Introduction_to_Organic_Synthesis.
- Bahl A. & Bahl B. S. (2006). A textbook of organic chemistry (for b. sc. students) (18th rev. & enlarged ed. 1st multicolor illustrative). S. Chand.